ToE Part II
PART II: Quantum Mechanics and Consciousness
Chapter 4: The Observer Effect and Wave Function Collapse
Review of the Measurement Problem in Quantum Mechanics
The measurement problem stands as one of the most profound and persistent puzzles in quantum mechanics, challenging our fundamental understanding of reality and the relationship between observers and the observed world. At its core, the measurement problem addresses a seemingly irreconcilable contradiction between two aspects of quantum theory: the deterministic evolution of quantum systems according to the Schrödinger equation and the probabilistic collapse that occurs during measurement.
When not being observed or measured, quantum systems evolve according to the Schrödinger equation, which describes how the wave function—a mathematical representation of all possible states of the system—changes over time. This evolution is smooth, deterministic, and linear, meaning that the system exists in a superposition of multiple possible states simultaneously. For example, an electron might exist in a superposition of different positions, energies, or spin states, with each possibility having a certain probability amplitude.
However, when a measurement is performed, something remarkable happens: the wave function appears to "collapse" instantaneously from a superposition of multiple possibilities to a single definite state. This collapse is probabilistic rather than deterministic—we can predict the probabilities of different outcomes but not which specific outcome will occur in any given measurement. This discontinuous, probabilistic collapse stands in stark contrast to the smooth, deterministic evolution described by the Schrödinger equation.
The measurement problem asks: How and why does this transition from multiple superposed possibilities to a single definite reality occur? What constitutes a "measurement," and what role, if any, does consciousness play in this process? These questions strike at the heart of our understanding of quantum reality and have profound implications for the nature of observation, consciousness, and physical reality itself.
Several major interpretations have been proposed to address the measurement problem, each with different implications for the relationship between consciousness and physical reality:
The Copenhagen Interpretation, developed primarily by Niels Bohr and Werner Heisenberg, maintains that quantum systems exist in superposition until measured, at which point they collapse probabilistically into definite states. This interpretation emphasizes the fundamental role of measurement in determining reality but remains somewhat ambiguous about the exact mechanism of collapse and whether consciousness plays any role in it. Bohr emphasized the inseparability of the quantum system from the measuring apparatus, suggesting that quantum properties only have meaning in the context of specific experimental arrangements.
The Many-Worlds Interpretation, proposed by Hugh Everett III, suggests that wave function collapse never actually occurs. Instead, all possibilities in the superposition are realized in different "branches" of reality that continuously split but cannot interact with each other. In this view, when we perceive a measurement outcome, we are simply in one branch of reality where that outcome occurred, while in other branches, other versions of ourselves observe different outcomes. This interpretation removes the special role of measurement and consciousness but requires the existence of countless parallel realities.
The Pilot Wave Theory, developed by Louis de Broglie and later expanded by David Bohm, proposes that particles always have definite positions and trajectories, guided by a "pilot wave" that evolves according to the Schrödinger equation. This interpretation maintains determinism at the expense of introducing non-local influences, as the pilot wave instantaneously affects particle behavior across space. Consciousness plays no special role in this interpretation, as particles always have definite properties regardless of observation.
The Objective Collapse Theories, such as the GRW (Ghirardi-Rimini-Weber) model, suggest that wave function collapse occurs spontaneously due to physical processes, independent of measurement or consciousness. These theories modify the Schrödinger equation to include non-linear terms that cause occasional spontaneous collapses, with the frequency of collapse increasing with the size of the system. This approach attempts to explain why quantum effects are not readily observable in macroscopic objects.
The Quantum Bayesianism (QBism) interpretation views the wave function not as a direct representation of physical reality but as a mathematical tool that encodes an observer's knowledge and expectations about a system. In this view, wave function collapse represents an update of knowledge rather than a physical process. While this interpretation places the observer at the center, it focuses on the observer's knowledge rather than consciousness per se.
The Von Neumann-Wigner Interpretation, proposed by John von Neumann and later advocated by Eugene Wigner, suggests that consciousness itself causes wave function collapse. This interpretation proposes that the chain of physical interactions in a measurement process must eventually terminate in consciousness, which is uniquely capable of collapsing the wave function. This perspective places consciousness in a central role in determining physical reality.
The Transactional Interpretation, developed by John Cramer, proposes that quantum events involve a "handshake" between retarded waves (moving forward in time) and advanced waves (moving backward in time). This handshake determines the outcome of quantum measurements without requiring consciousness or multiple worlds. This interpretation introduces a form of retrocausality that resonates with aspects of the Poia Theory.
The Relational Interpretation, proposed by Carlo Rovelli, suggests that quantum states are not absolute but exist only in relation to other systems. In this view, different observers may have different but equally valid descriptions of the same system, and there is no need for a universal collapse. This interpretation emphasizes the relational nature of physical properties without specifically invoking consciousness.
Each of these interpretations has strengths and limitations, and none has achieved universal acceptance among physicists. The persistence of multiple viable interpretations highlights the profound nature of the measurement problem and its resistance to resolution within conventional scientific frameworks.
Several key experiments have highlighted the reality and complexity of the measurement problem:
The Double-Slit Experiment demonstrates that particles such as electrons or photons exhibit wave-like behavior, creating interference patterns when passing through two slits, but particle-like behavior when observed at the slits. This experiment vividly illustrates how the act of observation appears to change the fundamental behavior of quantum systems.
The Delayed-Choice Experiment, proposed by John Wheeler and later implemented in various forms, shows that the decision to observe which path a particle takes can be made after the particle has already passed through the apparatus, yet still determines whether it behaved as a wave or particle earlier in its journey. This suggests that observation can influence the past, challenging conventional notions of causality.
The Quantum Eraser Experiment demonstrates that even when "which-path" information is recorded, erasing this information later can restore interference patterns, suggesting that the mere possibility of future knowledge affects present behavior. This experiment further complicates our understanding of measurement and its temporal implications.
The Stern-Gerlach Experiment shows that quantum properties like spin exist in superposition until measured, at which point they take on definite values. This experiment helped establish the probabilistic nature of quantum measurement and the reality of superposition states.
Bell Test Experiments have confirmed that quantum systems violate Bell's inequalities, demonstrating that quantum mechanics cannot be explained by local hidden variable theories. These experiments confirm the non-local nature of quantum reality, where measurement of one particle can instantaneously affect another particle regardless of distance.
These experiments and others have firmly established that the measurement problem is not merely a theoretical or philosophical concern but reflects genuine physical phenomena that require explanation. The act of measurement or observation genuinely appears to play a special role in determining quantum reality, though the exact nature of this role remains contested.
The Poia Theory of Everything approaches the measurement problem from a perspective that aligns most closely with the Von Neumann-Wigner interpretation but extends it in significant ways. Rather than seeing consciousness as merely collapsing an otherwise physical wave function, the Poia Theory proposes that consciousness is a fundamental field that interacts with the quantum field of potential (dark matter) through principles of resonance and frequency matching.
In the Poia framework, wave function collapse is not a mysterious exception to otherwise deterministic physical laws but a natural consequence of the interaction between consciousness and the field of potential. When consciousness observes or directs attention toward a quantum system, it resonates with specific frequencies within the superposition, amplifying those frequencies and increasing the probability of their manifestation. This resonant interaction explains why observation leads to collapse and why specific outcomes are selected with probabilities that match quantum mechanical predictions.
This perspective addresses several aspects of the measurement problem that other interpretations struggle with:
The Mechanism of Collapse: While many interpretations acknowledge that collapse occurs but provide no mechanism for it, the Poia Theory proposes resonance and frequency matching as specific mechanisms through which consciousness interacts with quantum fields to select particular outcomes from among possibilities.
The Role of the Observer: Rather than either eliminating the observer (as in Many-Worlds) or making consciousness mysteriously special without explanation (as in some versions of Copenhagen), the Poia Theory provides a framework for understanding how consciousness participates in physical reality through field interactions that follow consistent principles.
The Quantum-Classical Transition: The Poia Theory explains why quantum effects become less apparent at macroscopic scales, as larger systems involve more complex interactions with the consciousness field, leading to more stable and consistent outcomes through collective observation and resonance patterns.
Non-Locality and Entanglement: By understanding consciousness as a field phenomenon that operates non-locally, the Poia Theory provides a framework for understanding quantum non-locality and entanglement as manifestations of field interactions that transcend conventional spatial limitations.
Temporal Anomalies: The retrocausal aspects of quantum measurement, as demonstrated in delayed-choice experiments, align with the Poia understanding of time as non-linear and consciousness as potentially influencing systems across conventional temporal boundaries.
The measurement problem in quantum mechanics thus serves as both a challenge to conventional understanding and an opportunity for the Poia Theory to demonstrate its explanatory power. By proposing consciousness as a fundamental field that interacts with quantum systems through resonance and frequency matching, the Poia Theory offers a framework that addresses the measurement problem while integrating it into a broader understanding of the relationship between consciousness and physical reality.
This approach invites us to reconsider not only the specific puzzle of wave function collapse but our entire conception of the relationship between observers and the observed world. Rather than seeing ourselves as passive witnesses to an independent reality, the Poia Theory suggests that we are active participants in the ongoing creation of reality through the interaction of our consciousness with the field of potential. This participatory understanding has profound implications not only for physics but for our sense of agency, responsibility, and connection with the universe we inhabit.
Consciousness as the Collapse Mechanism
The Poia Theory of Everything proposes that consciousness serves as the primary mechanism for wave function collapse, transforming quantum possibilities into definite realities through its interaction with the field of potential. This perspective extends and refines the Von Neumann-Wigner interpretation of quantum mechanics, offering specific mechanisms through which consciousness might participate in the determination of physical reality.
At the heart of this proposal lies the understanding that consciousness is not merely an emergent property of complex physical systems but a fundamental field that permeates the universe. This consciousness field interacts with the quantum field of potential (dark matter) through principles of resonance and frequency matching, selectively amplifying certain possibilities within the superposition and increasing the probability of their manifestation as observable reality.
The process of wave function collapse through consciousness can be understood through several key principles:
Resonant Selection: When consciousness observes or directs attention toward a quantum system, it resonates with specific frequencies within the superposition that match or harmonize with its own vibrational qualities. This resonance creates a coupling between consciousness and those particular frequencies, amplifying them relative to other possibilities in the superposition.
Frequency Matching: The specific frequencies that consciousness resonates with depend on various factors, including the observer's state of awareness, intention, expectations, and past experiences. These factors create a unique "frequency signature" that determines which aspects of the quantum field the consciousness will most strongly couple with.
Coherent Amplification: As consciousness resonates with specific frequencies in the quantum field, it creates coherence among those frequencies, allowing them to work in phase and amplify each other. This coherent amplification increases the probability amplitude of the resonant frequencies, making their manifestation more likely during the collapse process.
Threshold Crossing: When the amplification of specific frequencies reaches a certain threshold, the wave function collapses, and one possibility from among the superposition becomes actualized as observable reality. This threshold represents the point at which the resonant coupling between consciousness and a particular potential becomes strong enough to select it definitively from among alternatives.
Information Integration: The collapse process involves the integration of information from the quantum system with the consciousness observing it, creating a unified experience that includes both the observer and the observed. This integration explains why measurement results appear consistent and reliable despite the probabilistic nature of quantum systems.
This understanding of consciousness as the collapse mechanism addresses several persistent puzzles in quantum mechanics:
The Measurement Problem: Rather than seeing wave function collapse as an unexplained exception to the Schrödinger equation, the Poia Theory positions it as a natural consequence of the interaction between consciousness and the quantum field. This interaction follows consistent principles of resonance and frequency matching, providing a coherent explanation for why and how collapse occurs.
The Preferred Basis Problem: Quantum systems can be described in multiple equivalent mathematical bases (position, momentum, energy, etc.), raising the question of which basis the wave function collapses in during measurement. The Poia Theory suggests that the basis of collapse is determined by the specific frequencies that consciousness resonates with, which depend on the nature of the observation being made and the apparatus being used.
The Probability Rule: Quantum mechanics accurately predicts the probabilities of different measurement outcomes using the Born rule, but does not explain why this particular mathematical rule applies. The Poia Theory suggests that these probabilities reflect the degree of resonance between consciousness and different frequencies within the superposition, with stronger resonance leading to higher probabilities of manifestation.
The Quantum-Classical Transition: The theory explains why quantum effects become less apparent at macroscopic scales, as larger systems involve interactions with multiple consciousnesses over time, creating more stable and consistent collapse patterns through collective observation. This collective resonance leads to the apparently deterministic behavior of classical systems despite their quantum underpinnings.
The role of consciousness in wave function collapse is not arbitrary or mysterious but follows principles that can be understood and potentially tested. Several specific aspects of this process deserve deeper exploration:
Intention and Attention: The Poia Theory suggests that both passive observation and active intention influence wave function collapse, but in somewhat different ways. Passive observation creates resonance based on the observer's existing state and expectations, while active intention involves deliberately focusing consciousness to resonate with specific desired outcomes. This distinction helps explain why quantum systems respond differently to different types of observation and why intention-based effects might be observable under certain conditions.
Individual and Collective Consciousness: While individual consciousness can collapse wave functions through personal observation, collective consciousness—the shared awareness of multiple observers—creates even stronger resonance patterns that lead to more stable and consistent collapse outcomes. This collective effect explains why publicly observed phenomena show such consistency despite the probabilistic nature of quantum systems and why scientific results can be reliably reproduced when multiple observers follow the same protocols.
Degrees of Collapse: The Poia Theory suggests that wave function collapse may not always be complete or absolute but can occur to varying degrees depending on the strength and coherence of the consciousness interacting with the quantum system. Weak or partial observation might lead to partial collapse, where some superposition remains, while strong, focused observation leads to more complete collapse into definite states. This perspective helps explain phenomena like the quantum Zeno effect, where frequent observation inhibits the evolution of a quantum system.
Non-Human Consciousness: If consciousness serves as the collapse mechanism, then the question arises of how quantum systems behave in the absence of human observers. The Poia Theory suggests that consciousness exists across a spectrum, with various degrees of awareness present throughout nature. Other animals, plants, and even simpler systems may participate in wave function collapse through their own forms of awareness, though perhaps with different qualities and effects than human consciousness. Additionally, the universal consciousness field itself may maintain a background level of observation that ensures reality maintains coherence even in the absence of specific observers.
Retrocausal Effects: Some quantum experiments, such as the delayed-choice quantum eraser, suggest that future observations can influence past events. The Poia Theory accommodates these findings by understanding consciousness as operating non-linearly with respect to time, potentially influencing quantum systems across conventional temporal boundaries. This perspective aligns with the concept of retrocausality, where future states can influence present conditions through attractive forces that guide systems toward specific potentials.
The proposal that consciousness serves as the collapse mechanism has profound implications for our understanding of reality and our place within it:
Participatory Universe: If consciousness participates in determining which quantum possibilities become actualized, then we are not passive observers of an independent reality but active participants in its ongoing creation. This participatory understanding aligns with John Wheeler's concept of the "participatory universe" and suggests a deeper level of engagement with reality than conventional scientific frameworks typically acknowledge.
Responsibility and Agency: The recognition that our consciousness influences physical reality through wave function collapse implies a level of responsibility for the reality we experience. While we cannot control all aspects of reality through conscious intention (due to the probabilistic nature of quantum systems and the influence of collective consciousness), we do participate in its creation in meaningful ways. This understanding invites a more intentional approach to how we direct our awareness and attention.
Scientific Objectivity: If consciousness influences measurement outcomes, then the ideal of a completely objective science independent of observers becomes problematic. However, the Poia Theory suggests that scientific objectivity emerges through collective observation following consistent protocols, which creates stable, reproducible results through shared resonance patterns. This perspective reframes objectivity not as the absence of observers but as the consistency of observations across multiple observers.
Technological Applications: Understanding consciousness as the collapse mechanism opens possibilities for technologies that work with this principle rather than ignoring or trying to eliminate it. Such technologies might enhance the coupling between consciousness and quantum systems for purposes such as healing, information processing, or energy generation. These applications would not violate quantum mechanical principles but would work with them in more sophisticated ways.
While the proposal that consciousness serves as the collapse mechanism extends beyond current scientific consensus, several lines of evidence provide support for this perspective:
Observer-Dependent Quantum Effects: Numerous quantum experiments demonstrate that the act of observation genuinely affects quantum systems, causing them to behave differently when observed versus unobserved. While conventional interpretations often try to explain these effects without invoking consciousness, the persistence and variety of observer-dependent phenomena suggest that observation plays a fundamental role in quantum reality.
Intention-Based Studies: Research in areas such as random number generator influence, distant mental interactions with living systems (DMILS), and intention-based healing has shown small but statistically significant effects that suggest consciousness may influence physical systems in ways aligned with the collapse mechanism proposed by the Poia Theory. While these studies remain controversial, meta-analyses have shown consistent effects across multiple laboratories and protocols.
Quantum Biology Findings: Emerging research in quantum biology has identified quantum coherence effects in biological systems that persist longer than conventional models would predict, suggesting that living systems may have evolved mechanisms to maintain and work with quantum superpositions. These findings align with the Poia understanding that consciousness, particularly as expressed through living systems, has a special relationship with quantum fields.
Phenomenological Reports: Practitioners of various consciousness disciplines consistently report experiences of directly influencing physical reality through focused awareness and intention. While subjective, the consistency of these reports across cultures and traditions suggests they may reflect genuine aspects of consciousness-reality interaction rather than mere illusion or suggestion.
The Poia Theory's proposal that consciousness serves as the collapse mechanism represents a significant departure from conventional scientific frameworks but offers a coherent explanation for numerous puzzling aspects of quantum mechanics. By positioning consciousness as a fundamental field that interacts with quantum systems through principles of resonance and frequency matching, the theory provides specific mechanisms for how observation might influence physical reality without requiring supernatural intervention or violations of physical law.
This perspective invites a reconsideration of the relationship between mind and matter, observer and observed, suggesting that these apparent dualities may be different aspects of a more fundamental unity. Rather than seeing consciousness as separate from or emergent from physical reality, the Poia Theory suggests that both consciousness and physical manifestation arise from the dynamic interplay of energy, frequency, and vibration within a unified field of potential.
The recognition of consciousness as the collapse mechanism thus represents not merely a solution to a specific problem in quantum mechanics but a fundamental shift in how we understand reality itself—a shift from a mechanistic universe that exists independently of observers to a participatory universe that continually emerges through the creative interaction of consciousness with the field of potential. This shift has profound implications not only for physics but for our understanding of ourselves as conscious beings and our role in the ongoing creation of reality.
Experimental Evidence for Observer-Dependent Reality
The proposition that reality is observer-dependent—that the act of observation genuinely influences what we perceive as real—stands as one of the most counterintuitive yet empirically supported aspects of quantum mechanics. The Poia Theory of Everything builds upon this evidence, suggesting that consciousness plays a fundamental role in determining which potentials within the quantum field become actualized as observable reality. A wealth of experimental findings provides support for this observer-dependent perspective, challenging conventional notions of an objective reality that exists independently of observation.
The Double-Slit Experiment and Its Variations
The double-slit experiment, first conducted with light by Thomas Young in 1801 and later extended to electrons and other particles, provides perhaps the most iconic demonstration of observer-dependent reality. In its quantum form, the experiment reveals that particles such as electrons or photons exhibit wave-like behavior, creating interference patterns when passing through two slits, but particle-like behavior when observed at the slits.
The basic experiment works as follows:
Particles (electrons, photons, etc.) are sent one at a time toward a barrier with two parallel slits.
When no observation is made to determine which slit each particle passes through, an interference pattern gradually builds up on the detection screen behind the slits, indicating wave-like behavior.
When detectors are placed at the slits to observe which path each particle takes, the interference pattern disappears, and two bands appear on the screen, indicating particle-like behavior.
This fundamental experiment has been refined and extended in numerous ways, each providing additional evidence for observer-dependent reality:
Quantum Erasure Variations: In these sophisticated versions, "which-path" information is recorded but then erased before the particle reaches the detection screen. Remarkably, when the information is erased, the interference pattern reappears, even though the particle has already passed through the apparatus. This suggests that it is not physical disturbance but information itself that determines whether wave or particle behavior manifests.
Delayed-Choice Variations: In these experiments, the decision to observe which path the particle takes is made after the particle has already passed through the slits but before it reaches the detection screen. Even with this delayed choice, the appropriate behavior (wave or particle) appears, suggesting that observation can influence the past state of the system.
Weak Measurement Variations: Using techniques that gather partial information without fully collapsing the wave function, researchers have observed intermediate states between wave and particle behavior. These experiments show that the transition from wave to particle is not all-or-nothing but can occur gradually depending on how much information is obtained, supporting the Poia concept of degrees of wave function collapse.
Quantum Entanglement Experiments
Experiments with entangled particles provide another powerful line of evidence for observer-dependent reality. When two particles are entangled, measuring a property of one particle instantaneously determines the corresponding property of the other, regardless of the distance separating them.
Bell Test Experiments: John Bell proposed inequalities that would be satisfied if reality were local and independent of observation. Numerous experiments, beginning with those of Alain Aspect in the 1980s and continuing with increasingly sophisticated designs, have violated Bell's inequalities, confirming that quantum systems cannot be described by local hidden variable theories. These results support the view that reality is not predetermined before observation but is genuinely influenced by the act of measurement.
Loophole-Free Bell Tests: Recent experiments have closed various "loopholes" that might have allowed for conventional explanations of earlier Bell test violations. These more rigorous tests, such as those conducted at Delft University in 2015, continue to show violations of Bell's inequalities, strengthening the case for observer-dependent reality.
GHZ Experiments: Tests with three or more entangled particles (Greenberger-Horne-Zeilinger states) show even stronger violations of local realism than two-particle entanglement, providing further evidence that reality is not independent of observation.
Quantum Zeno Effect
The quantum Zeno effect, named after the ancient Greek philosopher's paradox about motion, demonstrates that frequently observing a quantum system can inhibit its evolution. When a quantum system that would normally decay or change state is observed frequently enough, it becomes "frozen" in its initial state.
This effect has been demonstrated in various systems:
Trapped Ions: Experiments with beryllium ions have shown that their decay can be inhibited by frequent measurements.
Nuclear Spin Systems: NMR experiments have demonstrated the freezing of nuclear spin evolution through repeated observation.
Bose-Einstein Condensates: Studies have shown that the collapse of these quantum states can be delayed through continuous observation.
The quantum Zeno effect provides direct evidence that observation actively influences quantum systems rather than merely recording pre-existing states. This aligns with the Poia understanding of consciousness as actively participating in reality creation through wave function collapse.
Quantum Optics Experiments
Advanced experiments in quantum optics have provided additional evidence for observer-dependent reality:
Wheeler's Delayed-Choice Experiment: John Wheeler proposed a thought experiment where the decision to observe interference or which-path information is made after the photon has already "decided" whether to behave as a wave or particle. Actual implementations of this experiment, such as those by Jacques et al. (2007), confirm that the choice of measurement determines the photon's past behavior, challenging conventional notions of causality and supporting the view that reality is determined at the moment of observation rather than existing independently.
Quantum Teleportation: Experiments demonstrating quantum teleportation show that the quantum state of one particle can be transferred to another distant particle through entanglement and classical communication. These experiments highlight the non-local nature of quantum information and the role of measurement in actualizing specific states from among possibilities.
Hong-Ou-Mandel Effect: This quantum optical effect shows that identical photons arriving simultaneously at a beam splitter will always exit together rather than separately. This phenomenon demonstrates quantum interference at a fundamental level and has been used in various experiments that support the observer-dependent nature of quantum reality.
Quantum Biology Experiments
Emerging research in quantum biology provides evidence that quantum effects—including those that depend on observation—may play important roles in biological systems:
Photosynthetic Energy Transfer: Studies of light-harvesting complexes in plants and some bacteria have shown that energy transfer occurs through quantum coherence, with multiple pathways being explored simultaneously before the most efficient route is selected. This suggests that quantum superposition and collapse may be fundamental to biological processes.
Avian Magnetoreception: Research into how birds navigate using Earth's magnetic field suggests they may use quantum entanglement in their retinal proteins, with the coherence of these quantum states being maintained longer than conventional physics would predict in warm biological environments.
Olfaction Quantum Effects: Evidence suggests that smell may rely partly on quantum tunneling of electrons, with molecules being identified not just by their shape but by their quantum vibrational properties. This quantum aspect of a fundamental biological sense suggests deep connections between quantum reality and biological perception.
These biological findings suggest that living systems may have evolved to work with rather than against quantum effects, including observer-dependent phenomena, supporting the Poia view that consciousness and quantum fields are fundamentally interrelated.
Consciousness-Influenced Random Systems
Several lines of research have investigated whether human consciousness can directly influence random physical systems, providing potential evidence for consciousness-mediated wave function collapse:
Princeton Engineering Anomalies Research (PEAR): This long-running program at Princeton University conducted thousands of experiments testing whether human intention could influence the output of random event generators (REGs). Meta-analyses of these studies showed small but statistically significant deviations from chance expectation in the direction of participants' intentions.
Global Consciousness Project: This international collaboration maintains a network of random number generators around the world and analyzes whether their outputs show non-random patterns during major world events that capture widespread human attention. Analysis of data from over 500 events has shown small but statistically significant deviations from randomness, suggesting possible field effects of collective consciousness.
Psychokinesis Studies: Laboratory studies of micro-psychokinesis, where participants attempt to influence quantum-based random processes through intention, have shown small but consistent effects across multiple laboratories and protocols. Meta-analyses by researchers such as Dean Radin suggest these effects, while subtle, cannot be explained by chance or known methodological artifacts.
While these studies remain controversial within mainstream science, their consistent results across different laboratories, experimenters, and protocols provide suggestive evidence that consciousness may indeed influence quantum processes as proposed by the Poia Theory.
Quantum Computing Experiments
Research in quantum computing has provided additional evidence for the observer-dependent nature of quantum reality:
Quantum State Preparation: Creating and maintaining quantum states for computation requires careful control of observation and interaction, demonstrating in practical terms how measurement affects quantum systems.
Quantum Error Correction: Techniques for correcting errors in quantum computations often involve partial measurements that extract information about errors without fully collapsing the quantum state, illustrating the nuanced relationship between observation and wave function collapse.
Quantum Simulation: Quantum computers designed to simulate other quantum systems have confirmed many counterintuitive aspects of quantum mechanics, including observer effects, providing additional verification of these phenomena in controlled environments.
Near-Death Experience Research
While more controversial than laboratory physics experiments, research into near-death experiences (NDEs) provides another line of potential evidence for observer-dependent reality:
Veridical Perception Studies: Cases where individuals report accurate observations of their surroundings during periods when their brain activity was severely compromised suggest that consciousness may not be entirely dependent on brain function and might interact with reality in ways not limited by physical senses.
Shared Death Experiences: Documented cases where healthy individuals near a dying person report sharing aspects of the dying person's transition experience suggest consciousness may propagate beyond individual boundaries in ways that align with field models.
Cross-Cultural Consistency: The consistency of certain NDE features across different cultures and belief systems suggests they may reflect genuine aspects of consciousness-reality interaction rather than merely culturally constructed narratives.
While NDE research faces significant methodological challenges and alternative explanations exist for many reported phenomena, the accumulated evidence provides another perspective on potential consciousness-reality interactions that aligns with aspects of the Poia Theory.
Implications and Interpretation
The accumulated experimental evidence for observer-dependent reality strongly suggests that observation genuinely influences what we perceive as real, particularly at the quantum level. However, the interpretation of this evidence remains contested, with several perspectives offering different explanations:
Information-Based Interpretations suggest that it is not consciousness per se but information that determines quantum behavior. In this view, any process that extracts or records "which-path" information will cause wave function collapse, regardless of whether a conscious observer ever accesses that information.
Decoherence-Based Interpretations propose that interaction with the environment causes quantum systems to lose their wave-like properties through entanglement with numerous environmental degrees of freedom. In this view, apparent collapse occurs due to physical interactions rather than observation itself.
Consciousness-Based Interpretations, including the Poia Theory, suggest that consciousness plays a fundamental role in wave function collapse, selecting specific outcomes from among quantum possibilities through resonant interaction with the field of potential.
The Poia Theory integrates aspects of all these perspectives while emphasizing the role of consciousness. It suggests that:
Information is indeed crucial to wave function collapse, but information only exists in relation to consciousness that can potentially interpret it.
Decoherence plays an important role in the transition from quantum to classical behavior, but consciousness determines which decoherent state is actually experienced.
The observer effect operates through specific mechanisms of resonance and frequency matching rather than through mysterious or supernatural means.
This integrated perspective explains why observer effects are most evident in carefully controlled quantum experiments but less apparent in everyday experience. In daily life, objects interact with countless particles in their environment, creating extensive entanglement that leads to decoherence and apparent classical behavior. Additionally, collective observation over time creates stable patterns of wave function collapse that appear consistent and objective. However, at the quantum level or in systems partially isolated from environmental decoherence, the influence of consciousness becomes more evident.
The experimental evidence for observer-dependent reality provides strong support for key aspects of the Poia Theory of Everything, particularly its understanding of consciousness as actively participating in the creation of reality through wave function collapse. While alternative interpretations exist, the Poia framework offers a coherent explanation that integrates these findings into a comprehensive understanding of the relationship between consciousness and physical reality.
This evidence invites us to reconsider fundamental assumptions about the nature of reality and our place within it. Rather than seeing ourselves as passive observers of an independent world, we may be active participants in an ongoing process of reality creation, where consciousness and the physical world continuously interact through principles of resonance, frequency matching, and wave function collapse. This participatory understanding has profound implications not only for physics but for our approach to existence itself, suggesting a deeper level of engagement and responsibility in our relationship with reality.
Alternative Interpretations and Their Limitations
The observer effect and wave function collapse in quantum mechanics have generated numerous interpretations, each attempting to explain these phenomena within different philosophical and scientific frameworks. The Poia Theory of Everything offers its own distinctive interpretation, positioning consciousness as a fundamental field that interacts with quantum systems through resonance and frequency matching. To fully appreciate the Poia perspective, it is valuable to examine alternative interpretations and their limitations, highlighting where the Poia approach may offer advantages or insights that other interpretations lack.
The Copenhagen Interpretation
The Copenhagen Interpretation, developed primarily by Niels Bohr and Werner Heisenberg, was the first major attempt to make sense of quantum phenomena. It maintains that quantum systems exist in superposition until measured, at which point they collapse probabilistically into definite states according to Born's rule.
Key Features:
Emphasizes the fundamental role of measurement in determining reality
Maintains a distinction between the quantum and classical realms
Treats the wave function as a mathematical tool for calculating probabilities rather than a physical entity
Adopts a positivist approach that focuses on observable phenomena rather than underlying reality
Limitations:
Measurement Problem Unresolved: The Copenhagen Interpretation acknowledges that measurement causes wave function collapse but provides no mechanism for how or why this occurs, essentially treating collapse as an unexplained postulate of the theory.
Ambiguity About Consciousness: The interpretation remains ambiguous about whether consciousness plays any role in measurement, with different proponents taking different positions. This ambiguity leaves a central question unaddressed.
Arbitrary Quantum-Classical Boundary: The distinction between quantum and classical realms appears somewhat arbitrary, with no clear explanation for why different rules would apply at different scales.
Instrumentalist Approach: By focusing on measurement outcomes rather than underlying reality, the Copenhagen Interpretation has been criticized for being instrumentalist rather than realist, avoiding rather than solving the deeper ontological questions.
The Poia Theory addresses these limitations by proposing specific mechanisms (resonance and frequency matching) for how consciousness interacts with quantum systems, explaining the quantum-classical transition through collective observation and field effects, and offering a realist ontology where both consciousness and physical reality emerge from the interplay of energy, frequency, and vibration.
The Many-Worlds Interpretation
Proposed by Hugh Everett III, the Many-Worlds Interpretation suggests that wave function collapse never actually occurs. Instead, all possibilities in the superposition are realized in different "branches" of reality that continuously split but cannot interact with each other.
Key Features:
Preserves the deterministic evolution of the Schrödinger equation without collapse
Eliminates the special role of measurement and observers
Maintains a realist view of the wave function as physically real
Avoids the need for non-local influences or faster-than-light effects
Limitations:
Ontological Extravagance: The theory requires the existence of countless parallel realities that continuously branch but remain undetectable to each other, raising concerns about ontological parsimony.
Probability Problem: The theory struggles to derive the Born rule (which accurately predicts measurement probabilities) from first principles, requiring additional assumptions that some critics find ad hoc.
Preferred Basis Problem: Without collapse to select a specific measurement basis, the theory faces challenges in explaining why we experience the world in terms of specific observables (position, momentum, etc.) rather than arbitrary superpositions.
Consciousness Splitting: The theory implies that consciousness splits into multiple versions with each quantum event, raising profound questions about personal identity and experience that the interpretation does not fully address.
The Poia Theory avoids these limitations by maintaining wave function collapse as a real process while providing specific mechanisms for it, explaining probability distributions through resonance principles, addressing the preferred basis through consciousness-field interactions, and preserving the unity of individual consciousness while acknowledging its field-like properties.
The Pilot Wave Theory (Bohmian Mechanics)
Developed by Louis de Broglie and later expanded by David Bohm, this interpretation proposes that particles always have definite positions and trajectories, guided by a "pilot wave" that evolves according to the Schrödinger equation.
Key Features:
Maintains determinism at the quantum level
Particles have definite positions and trajectories at all times
The wave function serves as a "pilot wave" that guides particle motion
Measurement reveals pre-existing particle properties rather than creating them
Limitations:
Non-Locality: The theory requires instantaneous non-local influences, as the pilot wave must immediately affect distant particles. While quantum mechanics generally involves non-locality, Bohmian mechanics makes this feature particularly explicit and central.
Preferred Observable: The theory privileges position as the fundamental observable, with other properties being contextual, which some critics view as an arbitrary choice not justified by the mathematics.
Complexity in Many-Particle Systems: The mathematics becomes extremely complex for systems with many particles, requiring a 3N-dimensional configuration space for N particles, which makes practical calculations challenging.
No Role for Consciousness: The theory eliminates any special role for consciousness or observation, which conflicts with the apparent importance of measurement in quantum phenomena and fails to explain why observation appears to affect quantum systems.
The Poia Theory addresses these limitations by providing a framework for understanding non-locality through field effects, explaining the apparent importance of observation in quantum systems, and offering a more balanced treatment of different observables through the resonance principles that govern consciousness-field interactions.
Objective Collapse Theories
Theories such as the GRW (Ghirardi-Rimini-Weber) model suggest that wave function collapse occurs spontaneously due to physical processes, independent of measurement or consciousness.
Key Features:
Modifies the Schrödinger equation to include non-linear terms that cause occasional spontaneous collapses
Collapse frequency increases with system size, explaining why quantum effects are less evident in macroscopic objects
Maintains a realist view of the wave function while providing a physical mechanism for collapse
Eliminates the special role of observers
Limitations:
Parameter Fine-Tuning: These theories require precisely calibrated parameters to ensure that microscopic systems maintain quantum behavior while macroscopic systems collapse quickly enough to appear classical. This fine-tuning lacks independent justification.
Energy Non-Conservation: Some versions of these theories predict small violations of energy conservation due to the collapse process, which has not been observed despite sensitive tests.
Conflict with Relativity: Creating a relativistic version of these theories has proven challenging, as the collapse process seems to require a preferred reference frame, conflicting with the principles of relativity.
Experimental Evidence: Despite decades of searching, no direct evidence for the spontaneous collapse mechanisms proposed by these theories has been found, raising questions about their empirical support.
The Poia Theory avoids these limitations by not requiring modifications to the Schrödinger equation, maintaining consistency with energy conservation and relativity through field-based mechanisms, and aligning with experimental evidence for observer effects in quantum systems.
Quantum Bayesianism (QBism)
QBism views the wave function not as a direct representation of physical reality but as a mathematical tool that encodes an observer's knowledge and expectations about a system.
Key Features:
Wave function represents subjective knowledge rather than objective reality
Collapse represents an update of knowledge rather than a physical process
Emphasizes the personal, agent-centered nature of quantum theory
Avoids many traditional quantum paradoxes by treating quantum states as belief states
Limitations:
Subjectivist Approach: By treating quantum states as purely subjective, QBism has been criticized for being too instrumentalist and failing to address questions about the underlying reality that gives rise to our experiences.
Inter-subjective Agreement: The theory must explain why different observers tend to agree on measurement outcomes if quantum states are purely subjective, requiring additional assumptions about the nature of reality.
Physical Mechanisms: QBism focuses on the epistemological aspects of quantum mechanics (what we can know) while providing less insight into the ontological aspects (what exists), leaving questions about physical mechanisms largely unaddressed.
Consciousness-Reality Relationship: While placing the observer at the center, QBism focuses on the observer's knowledge rather than exploring how consciousness might interact with physical reality at a deeper level.
The Poia Theory addresses these limitations by providing an ontological framework that explains both subjective experience and physical reality through the interplay of energy, frequency, and vibration, while offering specific mechanisms for how consciousness interacts with quantum systems through resonance and field effects.
The Transactional Interpretation
Developed by John Cramer, this interpretation proposes that quantum events involve a "handshake" between retarded waves (moving forward in time) and advanced waves (moving backward in time).
Key Features:
Quantum events involve an exchange between emitters and absorbers
Includes both forward-in-time and backward-in-time influences
Provides a visual model for understanding quantum non-locality
Explains collapse without requiring consciousness or multiple worlds
Limitations:
Advanced Waves: The theory requires waves that travel backward in time, which, while mathematically consistent with quantum field theory, raises philosophical questions about causality and has no direct experimental confirmation.
Completion Challenge: The theory faces challenges in explaining how the "handshake" process is completed in cases where multiple potential absorbers exist, requiring additional assumptions about probability and selection.
Consciousness Question Unaddressed: While eliminating the need for consciousness in collapse, the theory does not explain why observation appears to play a special role in quantum phenomena or how conscious experience relates to quantum processes.
Complex Quantum Field Scenarios: The handshake mechanism becomes increasingly complex in scenarios involving quantum field theory and particle creation/annihilation, requiring elaborate extensions of the basic model.
The Poia Theory shares the Transactional Interpretation's recognition of non-linear temporality but addresses its limitations by providing a framework for understanding the apparent importance of observation in quantum systems and offering a more comprehensive account of the relationship between consciousness and physical reality.
The Relational Interpretation
Proposed by Carlo Rovelli, this interpretation suggests that quantum states are not absolute but exist only in relation to other systems.
Key Features:
Quantum states are relational rather than absolute
Different observers may have different but equally valid descriptions of the same system
No need for universal collapse or a privileged observer
Emphasizes the contextual nature of physical properties
Limitations:
Observer Definition: The theory must specify what constitutes an "observer" or "reference system" capable of having a perspective on quantum states, which risks either anthropocentrism or vagueness.
Consistency Conditions: The interpretation must explain why different observers' descriptions remain consistent with each other despite being relative, requiring additional assumptions about how perspectives relate.
Measurement Process: While avoiding universal collapse, the theory must still explain what happens during measurement from a given observer's perspective, essentially relocating rather than resolving the measurement problem.
Consciousness-Reality Relationship: The interpretation focuses on relational properties between physical systems but provides less insight into how consciousness relates to these physical interactions.
The Poia Theory addresses these limitations by offering a clear framework for understanding observers as systems capable of resonant interaction with the consciousness field, explaining consistency between observers through collective field effects, and providing specific mechanisms for the measurement process through resonance and frequency matching.
The Poia Interpretation: Advantages and Challenges
The Poia Theory of Everything offers its own distinctive interpretation of quantum phenomena, positioning consciousness as a fundamental field that interacts with quantum systems through resonance and frequency matching. This approach has several advantages over alternative interpretations:
Advantages:
Mechanism for Collapse: Unlike the Copenhagen Interpretation, the Poia Theory proposes specific mechanisms (resonance and frequency matching) for how consciousness interacts with quantum fields to select particular outcomes from among possibilities.
Integration of Observer: Rather than eliminating the observer (as in Many-Worlds) or treating observation as incidental (as in Bohmian mechanics), the Poia Theory provides a framework for understanding how consciousness participates in physical reality through field interactions that follow consistent principles.
Quantum-Classical Transition: The theory explains why quantum effects become less apparent at macroscopic scales, as larger systems involve more complex interactions with the consciousness field, leading to more stable and consistent outcomes through collective observation.
Non-Locality Explanation: By understanding consciousness as a field phenomenon that operates non-locally, the Poia Theory provides a framework for understanding quantum non-locality and entanglement as manifestations of field interactions that transcend conventional spatial limitations.
Temporal Anomalies: The retrocausal aspects of quantum measurement, as demonstrated in delayed-choice experiments, align with the Poia understanding of time as non-linear and consciousness as potentially influencing systems across conventional temporal boundaries.
Experiential Dimension: Unlike many interpretations that focus solely on mathematical formalism, the Poia Theory integrates the qualitative, experiential dimension of consciousness, addressing not just how quantum systems behave but how we experience them.
However, the Poia interpretation also faces several challenges:
Challenges:
Scientific Testability: Aspects of the theory involving consciousness-field interactions may be difficult to test using conventional scientific methods, requiring the development of new experimental approaches that can detect and measure these subtle interactions.
Mathematical Formalization: The resonance and frequency matching mechanisms proposed by the theory need more rigorous mathematical formalization to make precise, quantitative predictions that can be compared with experimental results.
Integration with Existing Physics: The theory must demonstrate how its consciousness field framework integrates with established physical theories, particularly quantum field theory and general relativity, without contradicting their well-verified predictions.
Parsimony Concerns: Critics may argue that introducing consciousness as a fundamental field adds unnecessary complexity compared to interpretations that explain quantum phenomena without reference to consciousness.
Definitional Clarity: The theory must provide clear, operational definitions of key concepts such as "consciousness," "resonance," and "frequency matching" to avoid vagueness or circularity in its explanations.
The Poia Theory acknowledges these challenges while suggesting that they represent opportunities for further development rather than insurmountable obstacles. By continuing to refine its conceptual framework, mathematical formalism, and experimental predictions, the theory aims to address these challenges while maintaining its distinctive perspective on the relationship between consciousness and quantum reality.
In conclusion, while alternative interpretations of quantum mechanics each offer valuable insights, they also face significant limitations in explaining the observer effect and wave function collapse. The Poia Theory of Everything provides a distinctive approach that addresses many of these limitations while offering a more comprehensive framework for understanding the relationship between consciousness and physical reality. By positioning consciousness as a fundamental field that interacts with quantum systems through principles of resonance and frequency matching, the Poia interpretation offers a path toward resolving longstanding puzzles in quantum mechanics while integrating the subjective dimension of experience that many other interpretations neglect or marginalize.
The Poia Interpretation of Quantum Measurement
The Poia Theory of Everything offers a distinctive interpretation of quantum measurement that positions consciousness as an active participant in the determination of physical reality through specific mechanisms of resonance and frequency matching. This interpretation addresses the measurement problem in quantum mechanics while integrating consciousness into a coherent framework that bridges subjective experience and objective reality.
At its foundation, the Poia interpretation understands quantum measurement not as the passive recording of pre-existing properties but as a dynamic interaction between consciousness and the quantum field of potential (dark matter). This interaction follows principles that are neither random nor arbitrary but governed by resonance relationships between the observer's consciousness and specific frequencies within the quantum superposition.
The Measurement Process in the Poia Framework
According to the Poia interpretation, quantum measurement unfolds through several interconnected stages:
1. Initial Superposition
Before measurement, a quantum system exists in a state of superposition described by its wave function—a mathematical representation of all possible states the system could occupy, each with its associated probability amplitude. This superposition is not merely a reflection of our ignorance about the system's "true" state but represents genuine ontological indeterminacy, with multiple potentials coexisting simultaneously.
In the Poia framework, this superposition corresponds to the quantum field of potential (dark matter), which contains all possible states in a form that has not yet been collapsed into definite reality. Each potential state within this field vibrates at its own characteristic frequency, creating a complex frequency spectrum that represents the system's full range of possibilities.
2. Consciousness-Field Interaction
When consciousness observes or directs attention toward the quantum system, it interacts with the field of potential through resonance. The observer's consciousness, which itself has specific frequency characteristics based on its state, expectations, intentions, and past experiences, resonates more strongly with certain frequencies within the superposition than with others.
This resonance is not random but follows principles similar to how a tuning fork vibrates sympathetically with sounds that match its natural frequency. The observer's consciousness naturally couples more strongly with frequencies in the quantum field that match or harmonize with its own vibrational qualities, creating a preferential amplification of those frequencies.
3. Resonant Amplification
As consciousness resonates with specific frequencies in the quantum field, it amplifies those frequencies through constructive interference, increasing their probability amplitude relative to other possibilities in the superposition. This amplification process is dynamic and can vary in strength depending on factors such as:
The intensity and focus of the observer's attention
The clarity and coherence of the observer's consciousness
The degree of match between the observer's frequencies and those in the quantum field
The presence of other resonant influences, including other observers or environmental factors
This amplification explains why quantum measurements yield results with probabilities that match the Born rule—the mathematical formula that relates the wave function to measurement probabilities. The probability of a particular outcome corresponds to the degree of resonance between the observer's consciousness and that potential state's frequency signature.
4. Threshold Crossing and Collapse
When the amplification of specific frequencies reaches a certain threshold, the wave function collapses, and one possibility from among the superposition becomes actualized as observable reality. This threshold represents the point at which the resonant coupling between consciousness and a particular potential becomes strong enough to select it definitively from among alternatives.
The collapse process is not instantaneous throughout space but propagates at the speed of light from the point of observation, explaining why distant entangled particles appear to be affected simultaneously from the perspective of external observers but without requiring faster-than-light communication.
5. Information Integration
The collapsed state becomes integrated into the observer's consciousness as information, creating a coherent experience that includes both the observer and the observed system. This integration explains why measurement results appear consistent and reliable despite the probabilistic nature of quantum systems.
The information from the measurement also becomes part of the observer's consciousness field, influencing its frequency characteristics and thereby affecting future interactions with quantum systems. This feedback loop creates continuity in experience while allowing for evolution and learning.
Key Features of the Poia Interpretation
Several distinctive features characterize the Poia interpretation of quantum measurement:
Consciousness as Field Phenomenon
Unlike interpretations that either eliminate consciousness or treat it as a mysterious exception to physical law, the Poia interpretation positions consciousness as a fundamental field that interacts with physical fields through principles of resonance and frequency matching. This field understanding explains how consciousness can influence quantum systems without requiring supernatural intervention or violations of physical principles.
The consciousness field is not separate from physical reality but represents a different aspect or expression of the same underlying trinity of energy, frequency, and vibration. This non-dual perspective avoids the problems of both dualism (how different substances interact) and materialism (how consciousness emerges from non-conscious matter).
Degrees of Collapse
The Poia interpretation suggests that wave function collapse may not always be complete or absolute but can occur to varying degrees depending on the strength and coherence of the consciousness interacting with the quantum system. Weak or partial observation might lead to partial collapse, where some superposition remains, while strong, focused observation leads to more complete collapse into definite states.
This perspective helps explain phenomena like the quantum Zeno effect, where frequent observation inhibits the evolution of a quantum system, and weak measurement techniques that extract partial information without fully collapsing the wave function. It also accounts for the gradual transition from quantum to classical behavior as systems interact with more observers and environmental factors.
Collective Observation Effects
While individual consciousness can collapse wave functions through personal observation, collective consciousness—the shared awareness of multiple observers—creates even stronger resonance patterns that lead to more stable and consistent collapse outcomes. This collective effect explains why publicly observed phenomena show such consistency despite the probabilistic nature of quantum systems and why scientific results can be reliably reproduced when multiple observers follow the same protocols.
The Poia interpretation suggests that what we experience as "objective reality" emerges from this collective observation process, where multiple consciousnesses resonate with similar frequencies in the quantum field, creating a shared reality through overlapping collapse patterns. This understanding bridges the apparent gap between the subjective nature of individual experience and the seeming objectivity of the physical world.
Non-Human Consciousness
If consciousness serves as the collapse mechanism, then the question arises of how quantum systems behave in the absence of human observers. The Poia interpretation suggests that consciousness exists across a spectrum, with various degrees of awareness present throughout nature. Other animals, plants, and even simpler systems may participate in wave function collapse through their own forms of awareness, though perhaps with different qualities and effects than human consciousness.
Additionally, the universal consciousness field itself may maintain a background level of observation that ensures reality maintains coherence even in the absence of specific observers. This perspective aligns with panpsychist approaches while providing more specific mechanisms for how consciousness at different levels interacts with physical systems.
Retrocausal Effects
Some quantum experiments, such as the delayed-choice quantum eraser, suggest that future observations can influence past events. The Poia interpretation accommodates these findings by understanding consciousness as operating non-linearly with respect to time, potentially influencing quantum systems across conventional temporal boundaries.
This perspective aligns with the concept of retrocausality, where future states can influence present conditions through attractive forces that guide systems toward specific potentials. Rather than seeing this as a violation of causality, the Poia interpretation suggests it reflects the more complex, non-linear nature of time and consciousness than conventional models assume.
Implications of the Poia Interpretation
The Poia interpretation of quantum measurement has profound implications for our understanding of reality and our place within it:
Participatory Universe
If consciousness participates in determining which quantum possibilities become actualized, then we are not passive observers of an independent reality but active participants in its ongoing creation. This participatory understanding aligns with John Wheeler's concept of the "participatory universe" and suggests a deeper level of engagement with reality than conventional scientific frameworks typically acknowledge.
This participation is not arbitrary or unconstrained but follows principles of resonance and coherence, explaining why reality appears stable and consistent despite its participatory nature. Our consciousness interacts with the quantum field according to patterns that reflect both our individual nature and our collective human experience, creating a reality that is neither purely subjective nor purely objective but emerges from the dynamic interplay between consciousness and the field of potential.
Responsibility and Agency
The recognition that our consciousness influences physical reality through quantum measurement implies a level of responsibility for the reality we experience. While we cannot control all aspects of reality through conscious intention (due to the probabilistic nature of quantum systems and the influence of collective consciousness), we do participate in its creation in meaningful ways.
This understanding invites a more intentional approach to how we direct our awareness and attention, recognizing that these subtle aspects of consciousness may have real effects on the physical world through resonant interaction with the quantum field. It suggests that practices that cultivate coherent, focused consciousness may enhance our capacity to influence reality in alignment with our intentions.
Scientific Objectivity Reconsidered
If consciousness influences measurement outcomes, then the ideal of a completely objective science independent of observers becomes problematic. However, the Poia interpretation suggests that scientific objectivity emerges through collective observation following consistent protocols, which creates stable, reproducible results through shared resonance patterns.
This perspective reframes objectivity not as the absence of observers but as the consistency of observations across multiple observers. It explains why scientific findings can be reliable and reproducible despite the observer-dependent nature of quantum reality, providing a framework for understanding science as a collective consciousness enterprise rather than the discovery of an observer-independent reality.
Technological Applications
Understanding quantum measurement as an interaction between consciousness and the quantum field opens possibilities for technologies that work with this principle rather than ignoring or trying to eliminate it. Such technologies might enhance the coupling between consciousness and quantum systems for purposes such as:
Information processing that leverages consciousness-quantum interactions
Healing modalities that work with resonance between consciousness and biological systems
Communication technologies that utilize non-local aspects of consciousness fields
Energy systems that tap into the quantum field of potential through resonant coupling
These applications would not violate quantum mechanical principles but would work with them in more sophisticated ways, recognizing consciousness as an integral part of the measurement process rather than an inconvenient complication to be minimized.
Experimental Support and Predictions
The Poia interpretation of quantum measurement makes several testable predictions that distinguish it from alternative interpretations:
1. Consciousness-Dependent Collapse Patterns
The interpretation predicts that the specific patterns of wave function collapse should correlate with the state and qualities of the observing consciousness. Different observers with different consciousness states (affected by factors such as meditation experience, emotional state, or intention) should show subtle but detectable differences in how they collapse identical quantum superpositions.
Experiments could test this by comparing collapse patterns across different observer states while controlling for other variables, potentially revealing correlations between consciousness qualities and quantum measurement outcomes.
2. Resonance Effects in Quantum Systems
The resonance mechanism proposed by the Poia interpretation suggests that quantum systems should show enhanced responsiveness to influences that match their natural frequencies. This predicts that intention-based effects on quantum systems should be stronger when the intention is "tuned" to resonate with the system's characteristics.
Experiments could test this by comparing the effects of differently "tuned" intentions on quantum random number generators or other quantum systems, looking for patterns that reflect resonance principles rather than simple deviation from randomness.
3. Collective Observation Amplification
The interpretation predicts that multiple observers focusing on the same quantum system should create stronger and more consistent collapse effects than individual observers, with the strength of the effect correlating with the coherence among the observers' intentions and attention.
Experiments could test this by comparing quantum measurement outcomes under conditions of individual observation versus coherent group observation, looking for evidence of amplified effects when multiple consciousnesses are aligned.
4. Partial Collapse Phenomena
The concept of degrees of collapse suggests that under certain conditions of partial or divided attention, quantum systems should show evidence of incomplete collapse, with some superposition remaining despite observation.
Experiments using weak measurement techniques could test for correlations between the degree of observer attention/focus and the completeness of wave function collapse, potentially revealing a spectrum of collapse states rather than an all-or-nothing phenomenon.
5. Retrocausal Influence Patterns
The interpretation's accommodation of retrocausal effects suggests that future observations should be able to influence quantum systems in the present in ways that correlate with the resonance between the observer's consciousness and specific potentials within the system.
Experiments could extend existing delayed-choice paradigms to include variations in observer state and intention, looking for patterns in how future observations affect present quantum states that align with resonance principles.
Challenges and Responses
The Poia interpretation of quantum measurement faces several significant challenges, which it addresses in specific ways:
Challenge 1: Scientific Testability
Critics argue that invoking consciousness in quantum measurement introduces elements that are difficult to test scientifically, potentially making the interpretation unfalsifiable.
Response: The Poia interpretation proposes specific mechanisms (resonance and frequency matching) and makes concrete predictions about how consciousness should interact with quantum systems under various conditions. These predictions can be tested through carefully designed experiments that vary observer states while measuring quantum outcomes. While challenging, such experiments are not impossible and represent an opportunity to extend scientific methodology rather than abandon it.
Challenge 2: Parsimony Concerns
Some critics suggest that introducing consciousness as a fundamental aspect of quantum measurement violates Occam's razor by adding unnecessary complexity compared to interpretations that explain quantum phenomena without reference to consciousness.
Response: The Poia interpretation argues that consciousness is not an additional element added to explain quantum measurement but a fundamental aspect of reality that cannot be eliminated from a complete understanding. Rather than creating additional complexity, recognizing the role of consciousness actually simplifies our understanding by providing a coherent explanation for phenomena that otherwise require multiple ad hoc assumptions or remain unexplained.
Challenge 3: Anthropocentrism
Critics worry that placing consciousness at the center of quantum measurement reintroduces an anthropocentric view that modern science has worked to overcome.
Response: The Poia interpretation is not anthropocentric, as it recognizes consciousness as a universal field that expresses through many systems beyond humans. It suggests that human consciousness is just one expression of a more fundamental field phenomenon that operates throughout nature, with other forms of awareness participating in wave function collapse according to their own characteristics. This perspective is actually less anthropocentric than interpretations that implicitly privilege human-scale classical reality.
Challenge 4: Quantum Field Theory Integration
Sophisticated quantum field theories describe particle interactions without explicitly invoking consciousness, raising questions about how the Poia interpretation integrates with these well-established frameworks.
Response: The Poia interpretation views quantum field theory as describing the mathematical structure and behavior of the quantum field of potential, while consciousness provides the mechanism by which specific potentials within this field become actualized through measurement. Rather than contradicting quantum field theory, the interpretation complements it by addressing aspects of measurement that the standard formalism acknowledges but does not explain.
In conclusion, the Poia interpretation of quantum measurement offers a coherent framework for understanding how consciousness participates in the determination of physical reality through resonant interaction with the quantum field of potential. By proposing specific mechanisms for this interaction and making testable predictions, the interpretation moves beyond philosophical speculation to engage with empirical science while addressing the profound questions raised by quantum measurement phenomena.
This interpretation invites us to reconsider not only the specific puzzle of wave function collapse but our entire conception of the relationship between observers and the observed world. Rather than seeing ourselves as passive witnesses to an independent reality, the Poia Theory suggests that we are active participants in the ongoing creation of reality through the interaction of our consciousness with the field of potential. This participatory understanding has profound implications not only for physics but for our sense of agency, responsibility, and connection with the universe we inhabit.
Chapter 5: Light as Presence
Reconceptualizing Light as Emanation Rather Than Travel
The Poia Theory of Everything proposes a fundamental reconceptualization of light that challenges our conventional understanding of how light moves through space and time. Rather than viewing light as traveling from one point to another, the theory suggests that light emanates from its source as an immediate presence, similar to how an electromagnetic field extends from its core. This perspective represents a significant departure from the standard model of light propagation and offers profound insights into the nature of light, presence, and the fabric of reality itself.
In the conventional understanding, light is described as electromagnetic waves or photon particles that travel through space at a constant speed (approximately 299,792,458 meters per second in vacuum). This model treats light as moving linearly from a source to a destination, taking time to traverse the distance between them. While this description has proven mathematically accurate for predicting light's behavior in various contexts, it leaves certain philosophical and conceptual questions unaddressed.
The Poia Theory challenges this conventional view by proposing that light does not "travel" in the ordinary sense but emanates from its source as an immediate presence. According to this perspective, when a light source is activated, light does not begin a journey from that point to other locations; rather, it becomes present throughout the space around the source, with its intensity diminishing with distance according to the inverse square law.
This reconceptualization can be understood through several key principles:
Emanation vs. Travel
In the emanation model, light is understood as a presence that extends outward from its source rather than as something that moves from one location to another. This presence is immediate within its sphere of influence, though the sphere itself expands at what we measure as the speed of light. The distinction is subtle but profound: in the conventional model, light is something that travels; in the emanation model, light is something that is present.
This perspective aligns with field concepts in physics, where electromagnetic fields are understood not as entities that travel but as conditions that exist throughout space, varying in intensity with distance from their source. Just as the gravitational field of the Earth doesn't travel to an apple but is already present at the apple's location, light can be understood as a field phenomenon that is present throughout its range rather than something that journeys from point to point.
The Speed of Presence
What we conventionally call the "speed of light" becomes, in this framework, the "speed of presence" or the "speed of emanation"—the rate at which the sphere of light's presence expands outward from its source. This speed represents not how fast light travels but how quickly the condition of "being illuminated" propagates through space.
This reconceptualization helps explain why the speed of light appears as a universal constant and a cosmic speed limit. It is not merely the velocity of a particular type of particle or wave but a fundamental property of how presence propagates through the fabric of reality. The speed of light thus represents the rate at which any form of presence, influence, or information can extend through space-time.
Removing the Time Component
One of the most significant aspects of this reconceptualization is how it transforms our understanding of light's relationship with time. In the conventional model, light requires time to travel from one point to another, creating a temporal gap between emission and reception. In the emanation model, this temporal component is fundamentally reframed.
Light is not moving from "then" to "now" but is present in an extended "now" that encompasses its entire sphere of influence. The apparent time delay we observe when light reaches us from distant objects represents not the travel time of light but the difference between when the sphere of presence began expanding from the source and when that expanding sphere reached our location.
This perspective aligns with certain interpretations of relativity that suggest the experience of time is relative and that what appears as temporal sequence from one reference frame may appear differently from another. The emanation model suggests that light exists in a kind of extended present that our consciousness, bound to a particular reference frame, perceives as temporal sequence.
Field Properties of Light
The emanation model emphasizes the field-like properties of light, viewing it as more similar to an electromagnetic field than to particles moving through space. Like a field, light:
Exists throughout its range simultaneously
Varies in intensity with distance from its source
Interacts with matter throughout its field of influence
Creates patterns of resonance and interference based on field interactions
This field perspective helps explain phenomena such as the wave-particle duality of light. Rather than being either particles traveling through space or waves propagating through a medium, light can be understood as a field phenomenon that interacts with matter in ways that sometimes appear particle-like and sometimes wave-like, depending on the nature of the interaction and observation.
Implications for Our Understanding of Reality
This reconceptualization of light as emanation rather than travel has profound implications for how we understand reality:
1. The Nature of Presence
If light emanates as presence rather than traveling as particles, this suggests that presence itself may be a more fundamental aspect of reality than we typically recognize. Presence—the quality of "being there"—may not be merely a subjective experience but a fundamental property of how energy expresses in space-time.
This understanding invites us to reconsider what it means for something to be present and how presence propagates and interacts across different domains of reality. It suggests that presence is not static but dynamic, not confined to points but extended through fields, not isolated but interconnected through resonance and coherence.
2. The Fabric of Space-Time
The emanation model suggests that space-time itself may be better understood as a field of potential presence rather than an empty container through which things travel. Space becomes not the absence between objects but the medium through which presence propagates and expresses.
This perspective aligns with certain interpretations of general relativity that view space-time not as an absolute background but as a relational field that both influences and is influenced by the energy and matter within it. The emanation of light becomes not movement through space but the expression of presence within the fabric of space itself.
3. The Observer's Perspective
The emanation model highlights the role of the observer in perceiving and interpreting light. What we experience as light traveling from distant objects to our eyes may be better understood as our consciousness intersecting with the field of light's presence that emanates from those objects.
This perspective suggests that observation is not merely the passive reception of light that has completed a journey but an active engagement with a field of presence that connects observer and observed. This understanding resonates with the Poia Theory's broader emphasis on the participatory nature of consciousness in shaping reality.
4. Quantum Entanglement and Non-Locality
The emanation model provides a conceptual framework for understanding quantum phenomena such as entanglement and non-locality. If light and other forms of energy are present throughout their sphere of influence rather than traveling point to point, then the apparent "spooky action at a distance" of quantum entanglement becomes less mysterious.
Entangled particles may be understood not as separate entities communicating faster than light but as expressions of a single field of presence that encompasses both particles. Changes in one region of this field are immediately present throughout the field, not because information travels between locations but because the field itself is a unified presence.
5. The Speed of Light as a Boundary Condition
In the emanation model, the speed of light represents not merely how fast light moves but a fundamental boundary condition of reality—the rate at which presence can propagate through the fabric of existence. This helps explain why this speed appears as a universal constant and cosmic speed limit.
Nothing can "travel" faster than light not because of limitations on velocity but because the concept of travel itself is secondary to the more fundamental process of presence propagation. The speed of light thus represents a basic property of how reality unfolds and extends through space-time.
Experimental Support and Predictions
While the emanation model represents a conceptual shift rather than a mathematical reformulation of existing physics, it does suggest certain experimental approaches and predictions:
1. Quantum Field Theory Alignment
The emanation model aligns with aspects of quantum field theory that describe particles as excitations in underlying fields rather than as discrete objects moving through space. Experiments exploring the field-like properties of light, such as those investigating quantum coherence and entanglement across extended regions, may provide insights relevant to the emanation perspective.
2. Quantum Eraser Experiments
The delayed-choice quantum eraser experiment, which suggests that future measurements can influence past light behavior, becomes more comprehensible in the emanation model. If light exists as a field of presence rather than particles traveling through time, then the apparent retrocausal effects may reflect the non-linear, field-like nature of light's presence rather than actual reverse causation.
3. Near-Field Optics
The behavior of light in the near field (very close to its source) often exhibits properties that are difficult to explain with conventional wave or particle models. The emanation perspective may provide a more intuitive framework for understanding these near-field phenomena, where light behaves more obviously as an extended field than as traveling rays.
4. Consciousness-Light Interactions
The emanation model suggests potential interactions between the field of light and the field of consciousness that might be experimentally explored. If both light and consciousness operate as fields of presence rather than localized phenomena, their interaction may exhibit properties that conventional models would not predict.
Philosophical and Practical Implications
Beyond its scientific significance, the reconceptualization of light as emanation rather than travel has profound philosophical and practical implications:
1. Metaphors for Understanding
The way we conceptualize fundamental phenomena like light shapes our broader understanding of reality. The shift from seeing light as something that travels to something that is present offers new metaphors for understanding other aspects of existence, from consciousness to information to influence.
These metaphors are not merely poetic but can guide scientific inquiry and technological innovation by suggesting new ways of approaching problems and possibilities. The emanation model invites us to think in terms of presence, fields, and resonance rather than particles, paths, and collisions.
2. Consciousness as Emanation
The Poia Theory draws explicit parallels between light and consciousness, suggesting that consciousness also emanates from individuals rather than being confined within them. Just as light extends as a field of presence from its source, consciousness may extend as a field of awareness from organisms with sufficient neural complexity.
This parallel provides a conceptual framework for understanding phenomena such as extended perception, collective consciousness, and non-local awareness that are difficult to account for in models that confine consciousness to the physical brain.
3. Technological Applications
The emanation perspective suggests potential technological approaches that work with light as a field of presence rather than as traveling particles or waves. These might include:
Holographic technologies that utilize the field properties of light more fully
Communication systems based on field resonance rather than signal transmission
Energy technologies that tap into the presence aspect of light rather than its particle or wave aspects
Consciousness technologies that explore the parallels between light fields and awareness fields
4. Spiritual and Contemplative Dimensions
The emanation model resonates with contemplative traditions that emphasize presence, radiance, and luminosity as fundamental aspects of both reality and consciousness. The understanding of light as presence rather than travel provides a conceptual bridge between scientific and spiritual perspectives on the nature of light, consciousness, and being.
This bridge invites a more integrated approach to knowledge that honors both the empirical precision of science and the experiential depth of contemplative wisdom, potentially enriching both domains through their creative interaction.
In conclusion, the Poia Theory's reconceptualization of light as emanation rather than travel represents a profound shift in how we understand one of the most fundamental phenomena in the universe. By viewing light as a presence that emanates from its source rather than as particles or waves that travel through space, the theory offers new insights into the nature of light, presence, and reality itself. This perspective challenges conventional assumptions while opening new possibilities for scientific exploration, technological innovation, and philosophical understanding. It invites us to see light not merely as something that illuminates the world but as a manifestation of presence itself—a fundamental quality of existence that connects all things in a luminous field of being.
The Speed of Light as the Speed of Presence
In the Poia Theory of Everything, the reconceptualization of light as emanation rather than travel leads to a profound reframing of what we conventionally call "the speed of light." Rather than representing how fast light particles or waves move through space, this universal constant is understood as the "speed of presence" or the "speed of emanation"—the rate at which the sphere of light's presence expands outward from its source. This perspective offers new insights into why this particular speed appears as a cosmic constant and how it relates to the fundamental nature of reality itself.
The speed of light (approximately 299,792,458 meters per second in vacuum) has several remarkable properties that have puzzled physicists since its measurement was refined in the late 19th and early 20th centuries:
It appears to be constant regardless of the motion of the source or observer, as confirmed by the Michelson-Morley experiment and numerous subsequent tests.
It represents an absolute cosmic speed limit that no material object or information can exceed, as established by Einstein's special relativity.
It connects seemingly disparate phenomena, appearing in equations related to electromagnetism, gravity, quantum mechanics, and other fundamental aspects of physics.
These properties suggest that the speed of light is not merely the velocity of a particular type of radiation but a fundamental constant woven into the fabric of reality itself. The Poia Theory's reframing of this speed as the "speed of presence" provides a conceptual framework for understanding why this constant holds such a central place in physics and how it relates to the nature of existence.
The Speed of Presence: A Fundamental Rate of Manifestation
In the emanation model, what we measure as the speed of light represents the fundamental rate at which presence propagates through the fabric of reality. This is not the velocity of something moving from point A to point B but the rate at which the condition of "being present" extends outward from a source.
This distinction is subtle but profound. When a light source is activated, it doesn't send particles or waves traveling outward that eventually reach distant points. Instead, it creates a sphere of presence that expands outward at what we measure as the speed of light. Within this expanding sphere, light is immediately present—not arriving after a journey but simply being there as the sphere encompasses new regions of space.
This perspective helps explain several puzzling aspects of the speed of light:
1. Reference Frame Independence
The constancy of the speed of light regardless of reference frame—one of the foundational principles of special relativity—becomes more intuitive in the presence model. If light doesn't travel in the conventional sense but represents an expanding sphere of presence, then its "speed" is not a velocity relative to anything else but a fundamental rate of presence propagation inherent to the fabric of reality itself.
This rate would naturally appear the same to all observers regardless of their motion, not because of strange relativistic effects (though these do occur) but because they are all measuring the same fundamental process—the rate at which presence propagates through existence. This rate is a property of reality itself rather than of objects moving through reality.
2. The Cosmic Speed Limit
The status of light speed as a universal speed limit also gains new meaning in this framework. Nothing can exceed the speed of light not because of some arbitrary restriction but because nothing can be present somewhere before presence itself has propagated to that location. The speed of light represents the fundamental "speed of becoming" in our universe—the rate at which anything can come to be present in a new location.
This perspective shifts our understanding from thinking of light speed as a limit on how fast things can travel to seeing it as a limit on how quickly presence can propagate. Material objects cannot exceed this speed not because of increasing mass or energy requirements (though these are the mathematical expressions of the limitation) but because their presence cannot extend faster than the fundamental rate at which presence itself propagates.
3. Dimensional Significance
The appearance of the speed of light in equations across different domains of physics reflects its status not as the velocity of a particular phenomenon but as a conversion factor between dimensions of reality. In the presence model, the speed of light represents the relationship between space and time—the rate at which presence extends through space as reality unfolds through time.
This dimensional significance explains why the speed of light appears in equations related to mass-energy equivalence (E=mc²), electromagnetic wave propagation, gravitational effects, and quantum field behavior. In each case, it serves as a fundamental conversion factor relating different aspects of reality, reflecting the underlying unity of these apparently diverse phenomena.
The Experience of Light Speed
The presence model also offers insights into how we experience and measure the speed of light:
1. Apparent Travel Time
When we observe light from distant objects, such as stars, we perceive a time delay that we conventionally interpret as the travel time of light from source to observer. In the presence model, this delay represents not the time light takes to travel but the difference between when the sphere of presence began expanding from the source and when that expanding sphere reached our location.
This reframing doesn't change the mathematical predictions or measurements but offers a different conceptual understanding of what those measurements represent. The light from a star 100 light-years away isn't 100-year-old light that has been traveling all that time; it's the presence of the star extending to us, with the 100-year delay reflecting when that presence began expanding rather than how long the journey took.
2. The "Now" of Light
From light's own perspective (if we could imagine such a thing), there is no experience of travel time—a photon emitted from a distant star would, in its own reference frame, be absorbed by an observer's eye instantaneously, with no time passing. This relativistic effect, often considered paradoxical in the conventional model, aligns naturally with the presence perspective.
If light represents presence rather than traveling particles, then from light's own perspective, it doesn't experience a journey through time but exists in an extended "now" that encompasses its entire sphere of influence. The temporal component that we perceive from our reference frame doesn't exist from the perspective of light itself.
3. Measurement Methodology
Our methods for measuring the speed of light typically involve timing how long it takes for light to traverse a known distance. In the presence model, what we're actually measuring is how quickly the sphere of light's presence expands to encompass new regions of space. The mathematical results remain the same, but the conceptual understanding of what those results represent shifts significantly.
This shift invites new approaches to thinking about light speed experiments, potentially suggesting methodologies that focus on the field properties of light rather than its particle or wave aspects. Such approaches might reveal subtleties in light's behavior that conventional models overlook.
Implications for Physics and Cosmology
The reconceptualization of light speed as the speed of presence has profound implications for various domains of physics and cosmology:
1. Relativity and Space-Time
Einstein's theories of relativity describe how space and time are not absolute but relative to the observer's reference frame, with the speed of light remaining constant for all observers. The presence model offers a conceptual framework for understanding why this is so: if the speed of light represents the fundamental rate at which presence propagates through reality, then it naturally appears constant regardless of reference frame.
This perspective suggests that the relativistic effects we observe—time dilation, length contraction, and the relativity of simultaneity—may reflect how different reference frames relate to the fundamental process of presence propagation. The mathematics of relativity remains unchanged, but its conceptual interpretation gains new dimensions.
2. Quantum Field Theory
In quantum field theory, particles are understood as excitations in underlying fields that permeate all of space. The presence model aligns with this field perspective, suggesting that light should be understood primarily as a field phenomenon rather than as traveling particles or waves.
This alignment invites deeper exploration of how the quantum field perspective relates to the concept of presence propagation. It suggests that quantum fields themselves may be understood as fields of potential presence, with particle-like behavior emerging when these fields interact with observing systems.
3. Cosmology and the Expanding Universe
The presence model offers interesting perspectives on cosmological phenomena such as the expansion of the universe. If the speed of light represents the rate at which presence propagates, then the expansion of the universe might be understood not merely as space stretching but as the fabric of presence itself extending.
This perspective could provide new conceptual approaches to puzzles such as dark energy, the accelerating expansion of the universe, and the nature of cosmic inflation. While not changing the mathematical models, it might suggest new ways of interpreting and exploring these phenomena.
4. The Nature of Time
Perhaps most profoundly, the presence model suggests a reconsideration of the nature of time itself. If light exists as presence rather than as something that travels through time, then time may be better understood as an aspect of how presence unfolds rather than as an independent dimension through which things move.
This perspective aligns with certain philosophical approaches to time that view it not as an absolute container but as a relational property emerging from the interactions and transformations of existence. The speed of light, as the speed of presence, might represent not how fast things move through time but how quickly the present moment itself unfolds and extends.
Practical and Technological Implications
Beyond its theoretical significance, the reconceptualization of light speed as the speed of presence suggests practical approaches and technological possibilities:
1. Communication Technologies
If light represents expanding presence rather than traveling signals, communication technologies might be developed that work with this presence aspect rather than treating signals as discrete packets moving through space. Such technologies might explore field resonance, quantum coherence, and other phenomena that leverage the presence properties of electromagnetic fields.
While these technologies would still be bound by the fundamental speed limit of presence propagation, they might achieve greater efficiency or capacity by working with rather than against the fundamental nature of light as presence.
2. Energy Technologies
The presence perspective suggests approaches to energy generation and transmission that focus on tapping into the field properties of light rather than its particle aspects. Technologies based on resonance, coherence, and field interactions might access aspects of light's energy that conventional approaches overlook.
These approaches would not violate energy conservation or other physical laws but might offer more elegant and efficient ways of working with electromagnetic energy by aligning with its fundamental nature as presence.
3. Measurement and Detection Systems
The presence model suggests new approaches to measuring and detecting light that focus on its field properties rather than treating it as discrete particles or waves. Such approaches might develop sensors that detect the presence aspect of light rather than counting photons or measuring wave intensities.
These detection systems might be particularly valuable for applications requiring sensitivity to subtle field effects or coherence properties that conventional detectors might miss.
Consciousness and the Speed of Presence
The Poia Theory draws explicit parallels between light and consciousness, suggesting that consciousness also emanates as a field of presence rather than being confined to specific locations. This parallel extends to the concept of the speed of presence:
1. Consciousness Propagation
Just as light's presence propagates outward at the speed of light, consciousness may extend outward from its sources at a rate governed by similar principles. This extension would not involve consciousness "traveling" from one location to another but would represent the expansion of a field of awareness that emanates from centers of neural complexity.
This perspective helps explain phenomena such as non-local awareness and extended perception, suggesting that consciousness naturally extends beyond the physical boundaries of the brain, creating a field of awareness that interacts with the environment and other consciousness fields.
2. Temporal Experience
The way we experience time may be intimately connected to the speed of presence. Our perception of events as occurring in sequence rather than simultaneously might reflect how our consciousness intersects with the expanding spheres of presence that constitute our reality.
This connection suggests that altered states of consciousness might involve changes in how we relate to the fundamental process of presence propagation, potentially explaining phenomena such as time dilation in meditative states or the feeling of timelessness in mystical experiences.
3. Collective Consciousness
If individual consciousness propagates as a field of presence, then collective consciousness might emerge from the interference patterns created when multiple consciousness fields overlap and interact. These interactions would be governed by principles similar to those that govern light field interactions, including resonance, coherence, and constructive/destructive interference.
This understanding provides a conceptual framework for phenomena such as group mind, collective intuition, and social synchronization that are difficult to explain in models that treat consciousness as confined to individual brains.
Philosophical and Spiritual Dimensions
The reconceptualization of light speed as the speed of presence resonates with various philosophical and spiritual traditions:
1. Presence as Fundamental
Many contemplative traditions emphasize presence as a fundamental aspect of reality and consciousness. The Buddhist concept of "suchness" (tathātā), the Taoist emphasis on being present with the flow of existence, and various mystical traditions' focus on the eternal now all align with the understanding of presence as more fundamental than movement through time.
The presence model provides a scientific framework that resonates with these traditions, suggesting that their emphasis on presence may reflect intuitive recognition of a fundamental aspect of reality that modern physics is only beginning to articulate.
2. Light as Metaphor and Reality
Light serves as a central metaphor in numerous spiritual traditions, symbolizing consciousness, divine presence, enlightenment, and ultimate reality. The presence model suggests that these metaphorical associations may reflect a deeper truth about the nature of light as presence rather than merely poetic analogies.
If light represents the propagation of presence itself, then its association with consciousness and divine reality in spiritual traditions may reflect an intuitive recognition of its fundamental role in the fabric of existence.
3. Unity of Being
The presence model suggests a fundamental unity underlying apparently separate phenomena, as both light and consciousness can be understood as expressions of presence propagating through the fabric of reality. This unity resonates with non-dual spiritual traditions that emphasize the underlying oneness of existence beneath the appearance of separation.
The speed of light, as the speed of presence, might represent not merely a physical constant but a fundamental aspect of how unity expresses as multiplicity through the propagation of presence across space and time.
In conclusion, the Poia Theory's reconceptualization of the speed of light as the speed of presence offers a profound shift in how we understand this fundamental constant. By viewing it not as the velocity of traveling particles or waves but as the rate at which presence propagates through the fabric of reality, the theory provides new insights into why this speed appears as a universal constant and cosmic speed limit.
This perspective does not contradict the mathematical formulations or experimental findings of modern physics but offers a different conceptual framework for interpreting and understanding them. It suggests that the speed of light represents something more fundamental than the velocity of a particular type of radiation—it represents the basic rate at which presence itself unfolds and extends through existence.
This understanding invites new approaches to theoretical physics, technological innovation, and the exploration of consciousness, while also creating bridges between scientific inquiry and contemplative wisdom traditions. By recognizing the speed of light as the speed of presence, we gain a more integrated understanding of this fundamental constant and its significance for our comprehension of reality itself.
Electromagnetic Fields and Consciousness Fields: Parallels and Differences
The Poia Theory of Everything draws compelling parallels between electromagnetic fields and consciousness fields, suggesting that both operate as fields of presence that emanate from their sources rather than as entities confined to specific locations. This comparison provides a powerful conceptual framework for understanding consciousness as a field phenomenon while also recognizing the unique qualities that distinguish consciousness fields from electromagnetic ones. Exploring these parallels and differences offers profound insights into the nature of both phenomena and their relationship to the fundamental fabric of reality.
Fundamental Parallels
Several key parallels between electromagnetic fields and consciousness fields form the foundation of this comparison:
1. Emanation from Sources
Both electromagnetic fields and consciousness fields emanate from their sources, extending outward to create spheres of influence that transcend the physical boundaries of those sources. Just as an electromagnetic field extends beyond the boundaries of a magnet or electrical conductor, consciousness extends beyond the physical boundaries of the brain, creating a field of awareness that interacts with the surrounding environment.
This emanation is not the movement of something from one location to another but the extension of presence outward from a center. The field is immediately present throughout its range, though its intensity typically diminishes with distance from the source according to specific mathematical relationships.
2. Field Properties
Both electromagnetic and consciousness fields exhibit characteristic field properties:
Superposition: Multiple fields can occupy the same space, combining their effects rather than excluding each other. Just as multiple electromagnetic fields can overlap and superimpose, multiple consciousness fields can coexist in the same space, creating complex patterns of interaction and influence.
Interference Patterns: When fields interact, they create interference patterns where their effects can either amplify (constructive interference) or cancel (destructive interference) each other. These patterns can be observed in both electromagnetic phenomena (such as light interference) and consciousness interactions (such as resonant or dissonant states between individuals).
Gradients of Intensity: Both fields typically exhibit gradients of intensity, being strongest near their sources and diminishing with distance according to specific patterns. However, these gradients are not always simple or linear, as fields can be focused, reflected, or otherwise modulated by various factors.
Non-Local Effects: Both fields can exhibit non-local effects, where changes in one region of the field can instantaneously affect distant regions without apparent signal transmission through the intervening space. This non-locality is well-established for quantum electromagnetic fields and suggested by various consciousness phenomena.
3. Resonance and Coherence
Both electromagnetic and consciousness fields operate through principles of resonance and coherence:
Resonant Coupling: Fields can couple with other systems that share similar frequency characteristics, transferring energy and information through resonance rather than direct contact. This principle underlies phenomena from radio transmission to empathic connection between individuals.
Coherence States: Both types of fields can achieve states of coherence where their oscillations become synchronized and aligned, creating more powerful and effective field effects. Laser light represents electromagnetic coherence, while states of focused meditation or group harmony represent consciousness coherence.
Harmonic Relationships: Both fields exhibit harmonic relationships, where frequencies related by simple mathematical ratios interact in particularly strong and stable ways. These harmonic principles underlie both electromagnetic phenomena like standing waves and consciousness experiences like musical harmony and aesthetic proportion.
4. Information Carrying Capacity
Both electromagnetic and consciousness fields serve as carriers of information:
Pattern Encoding: Both fields can encode complex patterns that carry information through variations in frequency, amplitude, phase relationships, and other parameters. These patterns can be transmitted, received, and interpreted by appropriate systems.
Bandwidth and Resolution: Both fields have characteristic bandwidth and resolution limitations that determine what types and quantities of information they can effectively carry. These limitations differ between the fields but reflect similar principles of information theory.
Signal-to-Noise Considerations: Both fields must contend with issues of signal clarity versus background noise, with various mechanisms for enhancing signal detection and interpretation in noisy environments.
5. Interaction with Matter
Both electromagnetic and consciousness fields interact with physical matter, though through different mechanisms:
Field-Matter Coupling: Both fields can couple with material systems, influencing their behavior and being influenced by them. These couplings follow principles of resonance, where systems that match the field's frequency characteristics interact most strongly.
Energy Transfer: Both fields can transfer energy to material systems, causing changes in their state or behavior. This energy transfer follows conservation principles but can take various forms depending on the nature of the interaction.
Information Exchange: Both fields can exchange information with material systems, either imprinting patterns onto the field or extracting patterns from it. This information exchange underlies phenomena from photography to memory formation.
Key Differences
Despite these compelling parallels, several important differences distinguish consciousness fields from electromagnetic fields:
1. Subjective Experience
The most fundamental difference is that consciousness fields incorporate subjective experience—the qualitative, first-person awareness that defines consciousness. While electromagnetic fields can be fully described in third-person, quantitative terms, consciousness fields inherently include the subjective dimension of what it feels like to be the system generating the field.
This subjective dimension has no clear analog in electromagnetic phenomena and represents a unique aspect of consciousness fields that extends beyond the parameters typically used to describe physical fields. It includes qualities such as:
The felt sense of being present and aware
The qualitative aspects of sensory experience (qualia)
The sense of self and agency
Emotional and affective states
Meaning and value dimensions
2. Intentionality and Agency
Consciousness fields exhibit intentionality—the capacity to be about or directed toward something beyond themselves. This intentional quality allows consciousness to focus attention, form intentions, and direct awareness in ways that have no direct parallel in electromagnetic fields.
This intentionality gives consciousness fields a degree of agency not present in electromagnetic fields. While electromagnetic fields follow deterministic laws of physics, consciousness fields can direct themselves through choices and intentions, creating patterns that are not merely the result of prior physical causes but reflect purposeful direction.
3. Self-Reflexivity
Consciousness fields possess self-reflexivity—the capacity to take themselves as objects of their own awareness. This creates the possibility for meta-awareness, self-reflection, and recursive levels of consciousness that have no clear analog in electromagnetic phenomena.
This self-reflexive quality allows consciousness fields to:
Monitor and modify their own states
Develop self-awareness and identity
Create recursive levels of awareness (being aware of being aware)
Engage in self-directed evolution and development
4. Meaning and Value Dimensions
Consciousness fields inherently incorporate dimensions of meaning and value that are absent from electromagnetic fields. While electromagnetic fields can carry information that humans interpret as meaningful, the meaning itself exists in the consciousness interpreting the information, not in the electromagnetic field.
Consciousness fields directly incorporate:
Semantic content and meaning
Valuation and preference
Ethical and aesthetic dimensions
Purpose and teleological aspects
These meaning and value dimensions are not secondary or derivative but intrinsic to the nature of consciousness fields, distinguishing them fundamentally from purely physical fields.
5. Evolutionary and Developmental Capacity
Consciousness fields demonstrate capacities for evolution and development that go beyond the changes observed in electromagnetic fields. While electromagnetic fields change in response to their sources and environments, consciousness fields can actively evolve through:
Learning from experience
Integrating new information and perspectives
Developing greater complexity and coherence
Expanding awareness and understanding
Cultivating specific qualities and capacities
This developmental capacity reflects the self-organizing and autopoietic (self-creating) nature of consciousness, which can actively participate in its own evolution rather than merely responding to external forces.
Interaction Between the Fields
The Poia Theory proposes that electromagnetic and consciousness fields are not entirely separate phenomena but interact in complex and significant ways: of Extended Consciousness**
The theory predicts that consciousness extends beyond the physical brain as a field with measurable properties:
Experimental Proposal: Use arrays of sensitive detectors (electromagnetic, quantum fluctuation, or other) to map the field around individuals in different consciousness states, looking for patterns that correlate with subjective reports and cannot be explained by conventional physical emissions.
Predicted Outcome: Detector arrays should reveal field patterns extending beyond the physical body that vary systematically with consciousness states and cannot be fully explained by known physical fields like electromagnetic radiation.
4. Collective Field Coherence Effects
The theory predicts that multiple consciousnesses focused on a common intention should create stronger field effects through constructive interference:
Experimental Proposal: Compare the effects of individual vs. group intention on quantum systems, with groups varying in size and degree of intentional coherence (from random individuals to highly trained meditation groups).
Predicted Outcome: Effects should scale non-linearly with group size when intentional coherence is high, demonstrating field-like interference patterns rather than simple additive effects.
5. Retrocausal Consciousness Effects
The theory predicts that consciousness may influence quantum systems across conventional temporal boundaries:
Experimental Proposal: Extend delayed-choice quantum experiments to include variations in observer state and intention, with the choice to observe made after the quantum event has already occurred according to conventional timelines.
Predicted Outcome: Observer state and intention should correlate with measurement outcomes even when the decision to observe is made after the quantum event, with the strength of correlation related to the coherence and focus of the observer's consciousness.
6. Dark Matter as Quantum Potential Field
The theory proposes that dark matter represents the physical manifestation of the quantum field of potential:
Experimental Proposal: Design experiments that look for correlations between quantum measurement outcomes and dark matter detection experiments, particularly during periods of focused collective attention on the experiments.
Predicted Outcome: Statistical patterns in quantum measurements should show correlations with dark matter detection events that cannot be explained by conventional physics, suggesting a deeper connection between these phenomena.
Challenges and Methodological Considerations
Testing these predictions faces several significant challenges that require careful methodological approaches:
1. Signal-to-Noise Ratio
The effects predicted by the theory may be subtle and easily obscured by noise or conventional explanations:
Methodological Approach: Use large sample sizes, sophisticated statistical analyses, and multiple independent replications to distinguish genuine effects from statistical artifacts or conventional explanations.
2. Observer Expectancy Effects
Conventional observer expectancy effects could be mistaken for the consciousness-field effects predicted by the theory:
Methodological Approach: Implement rigorous blinding procedures, automated data collection, and pre-registered analyses to minimize the influence of conventional psychological biases.
3. Replication Challenges
Consciousness-related effects may be sensitive to factors not typically controlled in physical experiments:
Methodological Approach: Develop standardized protocols that specify not only physical parameters but also consciousness-related variables such as observer state, intention quality, and environmental factors that might influence consciousness fields.
4. Theoretical Integration
Interpreting experimental results requires integrating findings across disciplines that often use different terminology and frameworks:
Methodological Approach: Develop interdisciplinary research teams and theoretical frameworks that can bridge physics, neuroscience, psychology, and consciousness studies, creating common language and concepts for interpreting results.
Existing Research Programs Aligned with Poia Predictions
Several existing research programs are investigating phenomena relevant to the Poia Theory's predictions:
1. Princeton Engineering Anomalies Research (PEAR) and Global Consciousness Project
These long-running programs have investigated mind-matter interactions and collective consciousness effects:
PEAR's decades of experiments showed small but consistent effects of human intention on random physical systems
The Global Consciousness Project has found correlations between major world events and non-random patterns in a global network of random number generators
These findings, while controversial, align with the Poia Theory's predictions about consciousness-field interactions with physical systems and collective consciousness effects.
2. Quantum Biology Research
Growing research into quantum effects in biological systems provides potential mechanisms for consciousness-field interactions:
Studies at institutions like the University of California, Berkeley and the University of Oxford are investigating quantum coherence in photosynthesis
Research at the University of Surrey and elsewhere is exploring quantum effects in neural systems
The Quantum Biology Laboratory at Howard University is investigating quantum processes in DNA and other biomolecules
These research programs are establishing that quantum effects can persist in biological systems despite warm, wet conditions, potentially providing mechanisms for consciousness-quantum field interactions.
3. Biofield Science Research
Various institutions are investigating the electromagnetic and other fields generated by living organisms:
The Consciousness and Healing Initiative (CHI) coordinates research into biofields and their healing applications
The Institute of Noetic Sciences conducts studies on biofield interactions and their relationship to consciousness
The Biofield Laboratory at the University of Arizona investigates measurable aspects of biofields and their effects
This research is developing methods for detecting and measuring fields associated with living systems that may correlate with consciousness fields as described in the Poia Theory.
4. Meditation and Consciousness Research
Numerous laboratories worldwide are studying the effects of meditation and other consciousness practices:
The Center for Healthy Minds at the University of Wisconsin-Madison studies how meditation affects brain function and extends to influence others
The Contemplative Sciences Center at the University of Virginia investigates how contemplative practices affect consciousness and its field-like properties
The Mind & Life Institute supports research into how meditation practices influence consciousness and its interaction with physical reality
These research programs are developing sophisticated methods for studying consciousness states and their effects, providing tools that could be applied to testing the Poia Theory's predictions.
Future Research Directions
Based on existing evidence and the predictions of the Poia Theory, several promising research directions emerge:
1. Quantum-Consciousness Interface Studies
Research focusing specifically on how consciousness interacts with quantum systems could test core predictions of the Poia Theory:
Experiments comparing quantum measurement outcomes across different consciousness states
Studies of how collective observation affects quantum coherence and decoherence
Investigations of resonance effects between brain states and quantum systems
These studies would directly address the theory's claims about consciousness as a collapse mechanism while potentially developing practical applications in quantum technologies.
2. Advanced Biofield Detection Methods
Developing more sensitive and specific methods for detecting and measuring biofields could provide direct evidence for consciousness fields:
Superconducting quantum interference devices (SQUIDs) for detecting subtle magnetic fields
Biophoton detection systems with single-photon sensitivity
Quantum sensors designed to detect field coherence rather than just field strength
Arrays of detectors that can map field patterns in three dimensions over time
These technologies could potentially provide direct measurement of consciousness fields, moving the theory from philosophical speculation to empirical science.
3. Collective Consciousness Networks
Large-scale networks for studying collective consciousness effects could test the field properties predicted by the theory:
Global networks of quantum random number generators synchronized with EEG measurements of participant groups
Coordinated meditation events with distributed measurement systems to detect field effects
Social network analyses combined with physiological measurements to study field propagation through human connections
These approaches could reveal how consciousness fields interact and combine across individuals, providing evidence for the field model while developing applications in social harmony and collective intelligence.
4. Dark Matter-Consciousness Correlation Studies
Research exploring potential connections between dark matter and consciousness could test one of the most distinctive predictions of the Poia Theory:
Experiments looking for correlations between dark matter detection events and consciousness measures
Studies examining whether collective meditation or intention practices affect dark matter detection rates
Theoretical models integrating quantum field theory, dark matter physics, and consciousness studies
While highly speculative, such research could potentially open entirely new domains of investigation at the intersection of cosmology and consciousness studies.
Conclusion: An Evidence-Based Approach to Revolutionary Concepts
The Poia Theory's reconceptualization of light as emanation and consciousness as a field phenomenon represents a potentially revolutionary shift in our understanding of fundamental reality. While aspects of the theory extend beyond current scientific consensus, numerous lines of evidence provide support for key elements of this framework, and specific experimental predictions offer paths for further investigation.
By generating testable predictions and engaging with existing research programs, the Poia Theory demonstrates that even revolutionary concepts can be approached through rigorous scientific methodology. The theory does not ask us to abandon empirical evidence but to expand our investigative methods to include consciousness as an active participant in physical reality rather than merely a passive observer or emergent property.
The evidence and predictions outlined here suggest that the Poia Theory, while challenging conventional frameworks, is not merely philosophical speculation but a potentially fruitful scientific approach to understanding the fundamental nature of light, consciousness, and their relationship to the fabric of reality. By pursuing the experimental proposals and research directions outlined above, we can determine whether this reconceptualization represents a genuine advance in our understanding of reality or merely an interesting but ultimately incorrect alternative to conventional models.
This evidence-based approach honors both the revolutionary potential of the Poia Theory and the empirical foundation of scientific inquiry, creating a path forward that neither dismisses unconventional ideas nor accepts them without rigorous testing. It is through this balanced approach that genuine scientific progress occurs, potentially leading to paradigm shifts that transform our understanding of reality and our place within it.
Light as a Metaphor and Literal Description of Consciousness
The Poia Theory of Everything proposes a profound connection between light and consciousness that operates simultaneously as both metaphor and literal description. This dual relationship illuminates the nature of consciousness while providing a conceptual bridge between scientific understanding and contemplative wisdom traditions. By exploring how light serves as both a metaphorical representation and a literal description of consciousness, we gain deeper insight into both phenomena and their fundamental relationship to reality itself.
Light as Metaphor for Consciousness
Throughout human history and across diverse cultures, light has served as a primary metaphor for consciousness, awareness, understanding, and spiritual awakening. This metaphorical connection appears in language, art, religious symbolism, and philosophical discourse:
1. Illumination and Understanding
The metaphor of light as understanding permeates language and thought:
We speak of "enlightenment" as the attainment of profound understanding
Ideas are described as "illuminating" when they create clarity
We have "flashes of insight" that suddenly illuminate a problem
To "shed light" on a topic means to make it comprehensible
"Brilliant" ideas shine with metaphorical light
This linguistic pattern reflects a deep intuition that consciousness and understanding operate like light, revealing what was previously hidden in darkness and making the world comprehensible.
2. Spiritual Awakening and Divine Presence
Across religious and spiritual traditions, light serves as the primary metaphor for spiritual consciousness and divine presence:
Buddhism speaks of "luminous mind" (pabhassara citta) as the natural state of consciousness
Christianity describes God as "light in whom there is no darkness" and spiritual transformation as moving from darkness to light
Hinduism uses light imagery extensively, from the inner light of Atman to the divine radiance of deities
Sufism employs the metaphor of the lamp and its light to describe the relationship between the individual and divine consciousness
Indigenous traditions worldwide associate spiritual power and awareness with the sun, fire, and other sources of light
This cross-cultural pattern suggests a universal human intuition that consciousness at its most fundamental level shares essential qualities with light.
3. Consciousness as Illumination of Reality
Philosophically, consciousness is often conceptualized as that which illuminates or reveals reality:
Phenomenology describes consciousness as that which discloses or reveals phenomena
The Indian philosophical tradition of Prakasha (light/illumination) views consciousness as self-luminous awareness that reveals objects
Western philosophical traditions speak of the "light of reason" that illuminates truth
Contemporary philosophy of mind often uses visual metaphors of "inner light" or "the mind's eye" to describe conscious awareness
These philosophical frameworks suggest that consciousness, like light, does not merely perceive reality but actively discloses or reveals it, making manifestation possible.
4. Qualities of Consciousness Metaphorically Associated with Light
Several specific qualities of consciousness find natural expression through light metaphors:
Clarity: Clear consciousness, like clear light, reveals objects distinctly and without distortion
Expansiveness: Consciousness, like light, can expand to fill a space without diminishing its source
Immediacy: Both light and consciousness operate with a sense of immediacy and presence
Gradations: Both can vary in intensity from dim awareness to brilliant clarity
Reflection: Both can be reflected, with consciousness reflecting on itself just as light reflects from surfaces
Pervasiveness: Both permeate their environment while remaining distinct from what they illuminate
These metaphorical connections are so pervasive and intuitive that they appear to reflect something fundamental about the nature of both light and consciousness rather than merely arbitrary cultural associations.
Light as Literal Description of Consciousness
Beyond metaphor, the Poia Theory proposes that light and consciousness share fundamental properties and mechanisms that make their relationship literal rather than merely symbolic. Several aspects of this literal relationship deserve exploration:
1. Field Properties
Both light and consciousness operate as fields that emanate from sources while extending beyond them:
Light emanates from sources as electromagnetic fields that extend through space
Consciousness emanates from organisms with sufficient neural complexity, creating fields of awareness that extend beyond physical boundaries
Both fields diminish in intensity with distance from their source while maintaining their essential qualities
Both fields can overlap and interact, creating interference patterns and resonance effects
Both fields transmit information through their patterns and modulations
These shared field properties suggest that the relationship between light and consciousness may be more than metaphorical—they may represent different expressions of similar underlying principles.
2. Wave-Particle Duality
Both light and consciousness exhibit characteristics of both waves and particles depending on how they are observed:
Light appears as waves in some experiments and as particles (photons) in others
Consciousness sometimes operates as a unified field (wave-like) and sometimes as discrete experiences or qualia (particle-like)
Both resist reduction to either waves or particles exclusively, suggesting a deeper reality that transcends this dichotomy
Both involve non-local effects that challenge conventional understandings of space and time
--
Chapter 6: Quantum Coherence and Microtubules
The Orch-OR Theory and its Relationship to Poia
The Orchestrated Objective Reduction (Orch-OR) theory, developed by physicist Sir Roger Penrose and anesthesiologist Stuart Hameroff, represents one of the most sophisticated scientific attempts to explain consciousness in terms of quantum processes in the brain. This theory provides a crucial foundation for the Poia Theory of Everything, which builds upon and extends Orch-OR's insights while integrating them into a broader framework of consciousness as a fundamental field phenomenon.
Foundations of the Orch-OR Theory
The Orch-OR theory emerged from the convergence of two distinct lines of inquiry: Penrose's mathematical exploration of consciousness and quantum gravity, and Hameroff's research into the potential quantum properties of microtubules in neurons. Their collaboration produced a theory that proposes specific physical mechanisms for how consciousness might arise from quantum processes in the brain.
Penrose's contribution began with his argument, articulated in "The Emperor's New Mind" (1989) and "Shadows of the Mind" (1994), that human understanding and consciousness involve non-computational processes that cannot be explained by classical physics or conventional artificial intelligence. He proposed that these non-computational aspects of consciousness must involve quantum mechanical processes, specifically a phenomenon he termed "objective reduction" (OR).
Objective reduction, in Penrose's framework, occurs when quantum superpositions reach a critical threshold related to quantum gravity, causing them to spontaneously collapse or "reduce" to definite states. Unlike standard interpretations of quantum mechanics that invoke measurement or observation to explain wave function collapse, Penrose's OR proposes that collapse occurs objectively due to instabilities in space-time geometry when quantum systems reach certain mass-energy thresholds.
Hameroff contributed the biological component to this theory through his research on microtubules—cylindrical protein structures that form part of the cytoskeleton in all eukaryotic cells, including neurons. Hameroff proposed that microtubules, composed of tubulin protein dimers arranged in lattice patterns, could potentially sustain quantum coherent states and serve as quantum processing units within neurons.
The synthesis of these ideas resulted in the Orch-OR theory, which proposes that:
Quantum computations occur in microtubules within neurons, specifically in the tubulin proteins that compose them
These quantum computations are "orchestrated" by synaptic inputs, memory, and various neurobiological processes
When these orchestrated quantum computations reach Penrose's threshold for objective reduction, consciousness occurs
The resulting moments of consciousness are linked together to create the continuous stream of awareness we experience
Key Components of Orch-OR
Several specific components of the Orch-OR theory are particularly relevant to the Poia framework:
Quantum Coherence in Microtubules: The theory proposes that tubulin proteins within microtubules can exist in quantum superposition states, where they essentially occupy multiple configurations simultaneously. These superpositions are maintained across networks of neurons through quantum coherence—a state where multiple quantum elements maintain phase relationships and act as a unified system.
Quantum Isolation Mechanisms: For quantum coherence to occur in the warm, wet environment of the brain, there must be mechanisms that protect quantum states from decoherence (the breakdown of quantum coherence through interaction with the environment). Orch-OR proposes several such mechanisms, including:
The ordered water within and surrounding microtubules, which may form quantum-isolating "Bose-Einstein condensates"
The periodic lattice structure of microtubules, which may create quantum channels protected from thermal disruption
Actin gelation, which may temporarily isolate portions of the cytoskeleton from environmental noise
Coherent oscillations that may help maintain quantum states through resonance effects
Objective Reduction: The theory proposes that when quantum coherent states in microtubules reach a specific threshold related to quantum gravity (the Penrose threshold), they spontaneously collapse or "reduce" to definite classical states. This objective reduction is proposed as the physical correlate of a conscious moment, with the specific pattern of the collapse determining the content of that conscious experience.
Temporal Binding: Orch-OR suggests that these quantum processes occur at extremely fast timescales (on the order of milliseconds or faster), with successive moments of objective reduction creating a sequence of conscious events that are bound together to form our continuous stream of awareness. This temporal binding helps explain how consciousness integrates information across different brain regions and sensory modalities.
Non-Locality: The theory incorporates quantum non-locality, suggesting that quantum coherence in the brain may enable consciousness to have properties that transcend conventional spatial limitations. This aspect of Orch-OR aligns with reports of non-local awareness in various altered states of consciousness.
How Poia Builds Upon and Extends Orch-OR
The Poia Theory of Everything incorporates the core insights of Orch-OR while extending them in several crucial ways:
1. From Production to Transduction
While Orch-OR generally frames consciousness as produced by quantum processes in the brain, the Poia Theory positions consciousness as a fundamental field that is transduced or channeled by these quantum processes rather than created by them. In this view, microtubules and their quantum properties serve not as generators of consciousness but as sophisticated antennas or receivers that couple with the consciousness field.
This shift from production to transduction resolves several challenges faced by Orch-OR:
It addresses the "hard problem" of consciousness by not requiring that subjective experience emerge from purely physical processes
It explains why consciousness appears to have properties (such as unity, intentionality, and subjectivity) that seem difficult to derive from physical systems alone
It accounts for phenomena suggesting consciousness may extend beyond the brain, such as near-death experiences and certain psi phenomena
It provides a framework for understanding how consciousness could interact with physical systems without violating physical laws
In the Poia framework, the quantum coherence in microtubules creates the conditions for resonant coupling with the consciousness field, with the specific patterns of this coherence determining which aspects of the field are expressed through the individual's experience.
2. Resonance as the Coupling Mechanism
The Poia Theory emphasizes resonance as the specific mechanism through which quantum systems in the brain couple with the consciousness field. This resonance occurs when the frequency patterns in neural microtubules match or harmonize with frequencies in the consciousness field, creating a preferential amplification of those frequencies.
This resonance framework extends Orch-OR by:
Providing a specific mechanism for how quantum systems in the brain interact with consciousness
Explaining why certain brain states correlate with specific consciousness states through frequency matching
Accounting for how consciousness might influence quantum collapse patterns through resonant coupling
Suggesting how practices like meditation might enhance this coupling by creating more coherent brain states
The resonance model also helps explain why consciousness appears to be associated primarily with certain types of complex systems (like brains) while still being a fundamental field—these systems have the specific organizational properties needed for strong resonant coupling with the consciousness field.
3. Dark Matter as the Quantum Field of Potential
While Orch-OR focuses primarily on quantum processes within the brain, the Poia Theory proposes a broader cosmological context by suggesting that dark matter represents the physical manifestation of the quantum field of potential—the field of all possibilities that consciousness interacts with through quantum processes.
This extension:
Connects neuroscience to cosmology, suggesting a fundamental relationship between consciousness and the structure of the universe
Provides a physical correlate for the field of potential that consciousness interacts with through quantum processes
Explains why quantum systems appear to exist in fields of probability until observed or measured
Suggests how consciousness might participate in reality creation through interaction with this field of potential
By identifying dark matter as the physical aspect of the quantum field of potential, the Poia Theory creates a framework where consciousness, quantum processes, and cosmology are integrated into a coherent whole.
4. Consciousness as a Spectrum
While Orch-OR tends to focus on human consciousness, the Poia Theory extends this to propose a spectrum of consciousness that spans from elementary awareness in simple systems to complex self-reflective consciousness in humans and potentially beyond.
This spectral view:
Explains how consciousness might be present to varying degrees throughout nature rather than suddenly emerging at a specific level of complexity
Accounts for the apparent continuity between human consciousness and other forms of awareness in the natural world
Suggests how consciousness might continue to evolve toward greater complexity and integration
Provides a framework for understanding altered states of consciousness as access to different portions of this spectrum
In this extended view, the quantum processes described by Orch-OR represent a particularly sophisticated form of coupling with the consciousness field, but not the only form—simpler quantum systems may couple with the field in less complex ways, creating simpler forms of awareness.
5. Non-Linear Temporality
The Poia Theory extends Orch-OR's treatment of time by proposing that consciousness operates non-linearly with respect to time, potentially influencing quantum systems across conventional temporal boundaries. This perspective builds on Penrose's insights about quantum gravity and time while extending them to include retrocausal effects.
This temporal extension:
Accounts for phenomena such as precognition and presentiment that suggest consciousness may access information across time
Explains how future states might influence present quantum processes through attractive potentials
Provides a framework for understanding how intention might influence quantum probability distributions across time
Suggests how consciousness might integrate information non-linearly to create meaningful patterns
By incorporating non-linear temporality, the Poia Theory creates a more comprehensive framework for understanding how consciousness interacts with quantum systems across both space and time.
6. Collective Consciousness Effects
While Orch-OR focuses primarily on individual consciousness, the Poia Theory extends this to include collective consciousness phenomena, where multiple individuals' quantum-consciousness interactions combine to create emergent field effects.
This collective dimension:
Explains how groups of individuals might create shared consciousness fields through resonant alignment
Accounts for social phenomena such as emotional contagion, crowd psychology, and collective intuition
Suggests how cultural and social fields might influence individual consciousness through field resonance
Provides a framework for understanding how collective intention might influence physical systems
By including collective consciousness effects, the Poia Theory creates a more socially and culturally embedded understanding of how quantum processes and consciousness interact.
Empirical Support and Challenges
Both Orch-OR and the Poia Theory face the challenge of empirical verification, though significant evidence supports aspects of both frameworks:
Supporting Evidence for Orch-OR:
Quantum Effects in Warm Systems: Research in quantum biology has demonstrated quantum coherence in photosynthesis, avian navigation, and other biological processes, suggesting that quantum effects can indeed persist in warm biological systems longer than previously thought possible.
Anesthetic Action on Tubulin: Studies have shown that anesthetic gases, which selectively and reversibly erase consciousness, primarily act on hydrophobic pockets in tubulin proteins—exactly where Orch-OR predicts quantum effects would occur.
Microtubule Vibrations: Measurements have confirmed that microtubules vibrate at specific frequencies in the megahertz to gigahertz range, as predicted by the Orch-OR model, potentially supporting quantum coherence.
Neuronal Dendritic-Somatic Integration: Evidence suggests that dendrites (where microtubules are particularly dense) perform complex processing rather than simply transmitting signals to the cell body, supporting the idea that significant information processing occurs in the cytoskeleton.
Gamma Synchrony Correlations: The 30-90 Hz gamma synchrony associated with consciousness correlates with the predicted frequencies of objective reduction events in the Orch-OR model.
Additional Support for Poia Extensions:
Field Effects of Consciousness: Studies suggesting non-local effects of consciousness, such as the Global Consciousness Project's findings of correlations between major world events and patterns in random number generators, support the field model of consciousness.
Dark Matter-Consciousness Correlations: While still speculative, certain anomalies in dark matter detection experiments have led some researchers to explore potential correlations with consciousness-related variables.
Resonance Effects in Brain States: Research on entrainment of brain states through rhythmic stimuli supports the resonance model of consciousness-brain interaction proposed by the Poia Theory.
Precognition Studies: Meta-analyses of presentiment and precognition experiments show small but statistically significant effects suggesting consciousness may indeed operate non-linearly with respect to time.
Collective Consciousness Phenomena: Studies of synchronization in groups, from fireflies to human brain waves in group meditation, support the concept of field-like collective consciousness effects.
Challenges and Criticisms:
Both theories face significant challenges and criticisms from mainstream science:
Quantum Decoherence Concerns: Critics argue that quantum coherence cannot be maintained long enough in the warm, wet brain environment for the proposed effects to occur, though proponents counter with specific isolation mechanisms and evidence from quantum biology.
Measurement Difficulties: Directly measuring quantum effects in living neural tissue presents enormous technical challenges, making empirical verification difficult.
Explanatory Gap: Some critics argue that even if quantum processes occur in the brain, they don't explain how or why consciousness arises, though the Poia framework addresses this by positioning consciousness as fundamental rather than emergent.
Parsimony Concerns: Critics suggest that simpler, classical explanations of neural function should be preferred over quantum models, though proponents argue that classical models have failed to explain key features of consciousness.
Integration with Mainstream Neuroscience: Both theories face challenges in integrating their proposals with the vast body of knowledge from conventional neuroscience, though efforts to bridge this gap continue.
Theoretical and Practical Implications
The integration of Orch-OR within the broader Poia framework has significant theoretical and practical implications:
Theoretical Implications:
Mind-Matter Relationship: The combined framework suggests a more integrated relationship between mind and matter, where consciousness is neither reduced to physical processes nor completely separate from them but interacts with physical systems through specific quantum mechanisms.
Evolution of Consciousness: The framework suggests that the evolution of increasingly complex nervous systems represents the development of more sophisticated quantum transducers for the consciousness field rather than the emergence of consciousness itself from non-conscious matter.
Free Will and Agency: The quantum indeterminacy at the heart of both theories provides a potential physical basis for genuine choice and agency, suggesting how consciousness might influence physical systems without violating physical laws.
The Nature of Reality: The integrated framework suggests a participatory universe where consciousness plays an active role in determining which potentials become actualized through its interaction with the quantum field of potential.
Practical Implications:
Medical Applications: Understanding consciousness as interacting with the brain through quantum processes suggests new approaches to treating disorders of consciousness, developing more effective anesthetics, and potentially even enhancing consciousness.
Technological Development: The framework suggests possibilities for technologies that might detect, measure, or even interact with the consciousness field through quantum interfaces.
Consciousness Practices: The resonance model provides a theoretical foundation for practices like meditation, suggesting specific mechanisms by which they might enhance the coupling between brain states and the consciousness field.
Social and Environmental Applications: The collective consciousness aspects of the Poia framework suggest approaches to enhancing group coherence, resolving conflicts, and potentially even addressing environmental challenges through collective intention.
Conclusion: A Bridge Between Physics and Consciousness
The relationship between the Orch-OR theory and the Poia Theory of Everything represents a promising bridge between rigorous physics and the study of consciousness. By incorporating Orch-OR's detailed quantum mechanisms within a broader framework that positions consciousness as a fundamental field, the Poia Theory creates a more comprehensive approach that addresses both the "how" and the "why" of consciousness.
This integration suggests that consciousness is neither a mysterious force outside the physical world nor merely an emergent property of complex neural activity, but a fundamental aspect of reality that interacts with physical systems through specific quantum mechanisms. The microtubules within neurons, with their remarkable quantum properties, serve as sophisticated transducers that couple with this consciousness field, creating the rich, unified experience of being that defines our existence.
As research in quantum biology, neuroscience, and consciousness studies continues to advance, this integrated framework offers a roadmap for exploring the profound relationship between quantum physics, biology, and consciousness—a relationship that may ultimately reveal consciousness not as an anomaly in an otherwise unconscious universe but as an integral aspect of reality itself, expressed through but not limited to the remarkable quantum processes occurring in our brains.
Quantum Processes in Biological Systems
The Poia Theory of Everything builds upon emerging research in quantum biology—a field that explores how quantum mechanical phenomena may play crucial roles in biological processes. This research challenges the conventional assumption that quantum effects are irrelevant to biology due to the warm, wet, and complex nature of living systems. Instead, it suggests that certain biological processes not only tolerate quantum effects but may have evolved specifically to harness them. Understanding these quantum biological processes provides essential context for the Poia Theory's proposals about consciousness-matter interactions and the quantum foundations of consciousness itself.
Fundamental Quantum Effects in Biology
Several quantum mechanical phenomena appear to play significant roles in biological systems:
1. Quantum Tunneling
Quantum tunneling occurs when particles pass through energy barriers that would be insurmountable according to classical physics. This phenomenon appears to be crucial in several biological processes:
Enzyme Catalysis: Research suggests that proton and electron tunneling significantly enhances the rate of certain enzymatic reactions. For example, the enzyme soybean lipoxygenase-1 shows reaction rates 1000 times faster than would be possible without quantum tunneling. Studies by Judith Klinman at UC Berkeley and others have demonstrated that tunneling allows hydrogen atoms to move through energy barriers during catalysis, dramatically increasing reaction efficiency.
DNA Mutation: Quantum tunneling may contribute to spontaneous mutations in DNA when protons tunnel between complementary base pairs, temporarily altering their bonding patterns. This quantum effect could explain certain aspects of genetic variation that classical models struggle to account for.
Cellular Respiration: The electron transport chain in mitochondria, which is fundamental to cellular energy production, appears to utilize quantum tunneling to move electrons between protein complexes more efficiently than classical physics would allow.
2. Quantum Coherence and Superposition
Quantum coherence occurs when particles maintain phase relationships, allowing them to exist in multiple states simultaneously (superposition) and act as unified systems:
Photosynthetic Energy Transfer: Perhaps the most well-established example of quantum coherence in biology involves the remarkable efficiency of photosynthesis. Research by Graham Fleming at UC Berkeley and teams at the University of Toronto and elsewhere has shown that energy captured from photons appears to travel as a coherent quantum wave rather than a particle, simultaneously exploring multiple pathways to find the most efficient route to reaction centers. This quantum "wavelike energy transfer" explains how plants and certain bacteria achieve near 100% efficiency in converting photons to chemical energy under ideal conditions.
Magnetoreception in Birds: Evidence suggests that migratory birds navigate using Earth's magnetic field through a quantum coherent process involving cryptochrome proteins in their retinas. When light strikes these proteins, it creates quantum entangled radical pairs whose spin states are sensitive to magnetic fields. Research by teams at Oxford University and elsewhere has shown that this quantum mechanism can detect both the intensity and direction of magnetic fields, providing birds with a literal "quantum compass."
Olfaction (Smell): The "vibrational theory" of olfaction, championed by Luca Turin, proposes that our sense of smell relies partly on quantum tunneling of electrons that are sensitive to the vibrational frequencies of molecules rather than just their shape. This quantum mechanism would explain how we can distinguish between molecules with identical shapes but different isotopes (and thus different vibrational frequencies).
3. Quantum Entanglement
Quantum entanglement occurs when particles become correlated in ways that cannot be explained by classical physics, with the state of one particle instantaneously influencing another regardless of distance:
Photosynthetic Reaction Centers: Research suggests that electronic excitations in photosynthetic reaction centers may exhibit quantum entanglement, allowing for more efficient energy transfer and charge separation. Studies using two-dimensional electronic spectroscopy have detected signatures consistent with quantum entanglement in these systems.
Avian Magnetoreception: The radical pair mechanism in bird navigation appears to rely on quantum entanglement between electrons, with the entangled spin states providing heightened sensitivity to magnetic fields. This entanglement is maintained long enough to influence biochemical reactions that ultimately affect the bird's perception.
Enzymatic Catalysis: Some research suggests that quantum entanglement between electrons in enzymatic reactions may contribute to the remarkable efficiency and specificity of certain enzymes. This entanglement could allow for coordinated electron movements that facilitate complex chemical transformations.
4. Zero-Point Energy Effects
Zero-point energy—the energy that remains in quantum systems even at absolute zero temperature—may influence biological processes:
Protein Folding: The precise folding of proteins into their functional three-dimensional structures may be influenced by zero-point energy fluctuations that help overcome energy barriers between different conformational states.
Molecular Recognition: The specific binding between molecules, such as enzymes and substrates or antibodies and antigens, may be enhanced by zero-point energy effects that contribute to the energetics and specificity of these interactions.
Membrane Dynamics: Zero-point fluctuations may influence the behavior of cell membranes, potentially affecting permeability, fluidity, and the function of membrane-embedded proteins.
Quantum Processes in Neural Systems
Of particular relevance to the Poia Theory are quantum processes that may occur specifically in neural systems, potentially creating interfaces between quantum fields and consciousness:
1. Microtubule Quantum Effects
Microtubules—cylindrical protein structures that form part of the cytoskeleton in all eukaryotic cells, including neurons—have been proposed as potential quantum processing structures:
Quantum Oscillations: Research by Anirban Bandyopadhyay at the National Institute for Materials Science in Japan has measured quantum resonance patterns in microtubules at multiple frequencies, from kilohertz to gigahertz ranges. These resonances create coherent oscillations that could potentially sustain quantum states.
Ordered Water: The interior of microtubules contains highly ordered water molecules that may form quantum-coherent structures similar to Bose-Einstein condensates. This ordered water could provide quantum isolation from the surrounding cellular environment, protecting quantum states from decoherence.
Aromatic Rings: The tubulin proteins that make up microtubules contain networks of aromatic amino acids (tryptophan, tyrosine, phenylalanine) whose π-electron clouds may support quantum effects such as superposition and entanglement. These aromatic networks are particularly concentrated in the hydrophobic pockets where anesthetic gases bind—precisely the sites that selectively and reversibly erase consciousness.
Topological Quantum Computing: The lattice structure of microtubules, with its specific geometry and connectivity patterns, may support topological quantum computing—a form of quantum information processing that is inherently more stable against decoherence than conventional quantum computing approaches.
2. Neural Quantum Communication
Beyond individual microtubules, quantum effects may facilitate communication between neurons:
Biophoton Signaling: All living cells, including neurons, emit ultra-weak photon emissions (biophotons) that appear to be coherent. Research by Fritz-Albert Popp and more recently by teams at several institutions suggests these biophotons may play a role in cell-to-cell communication. In neural tissue, these quantum-coherent photons could potentially carry information between neurons through quantum optical effects.
Quantum Tunneling in Synapses: Some research suggests that neurotransmitter release at synapses may be influenced by quantum tunneling effects, potentially introducing quantum indeterminacy into neural signaling. This quantum component could help explain why synaptic transmission shows probabilistic rather than deterministic behavior.
Gap Junction Quantum Effects: Electrical synapses formed by gap junctions directly connect the cytoplasm of adjacent cells and may provide channels for quantum coherence to extend across multiple neurons, potentially creating larger-scale quantum networks within the brain.
3. Electromagnetic Field Effects
The electromagnetic fields generated by neural activity may interact with quantum processes in ways relevant to consciousness:
Quantum Field Interactions: The electromagnetic fields generated by synchronized neural activity may interact with quantum fields in ways that influence quantum coherence and entanglement in neural structures. These interactions could potentially create feedback loops between classical electromagnetic activity and quantum processes.
Spin Dynamics: The electromagnetic fields in the brain may influence the spin states of electrons and atomic nuclei in neural tissue, potentially affecting quantum processes that depend on spin coherence, such as those proposed in quantum models of consciousness.
Josephson Junctions: Some researchers have proposed that structures in the brain may function similarly to Josephson junctions—quantum devices where current flows through a thin insulating barrier via quantum tunneling. These biological "Josephson junctions" could potentially couple electromagnetic fields to quantum processes.
Mechanisms for Maintaining Quantum Coherence
A key challenge for quantum biology is explaining how quantum coherence can be maintained in the warm, wet environment of living cells, where conventional physics would predict rapid decoherence. Several mechanisms have been proposed and are finding experimental support:
1. Environmental Noise Assistance
Counterintuitively, certain types of environmental noise may actually enhance rather than destroy quantum coherence in biological systems:
Noise-Assisted Quantum Transport: Research on photosynthetic complexes suggests that the specific "noise" of cellular environments—the pattern of vibrations and fluctuations—may actually help maintain quantum coherence through a phenomenon called environment-assisted quantum transport (ENAQT). Rather than viewing the cellular environment as disrupting quantum effects, this perspective sees the environment as having co-evolved with quantum systems to protect and enhance quantum coherence.
Vibronic Coupling: Studies have shown that specific vibrational modes of proteins can couple with electronic quantum states, protecting them from decoherence and even enhancing quantum effects such as tunneling and coherent energy transfer. This vibronic coupling (the interaction of vibrational and electronic states) may represent an evolved mechanism for harnessing quantum effects.
2. Topological Protection
The specific structural arrangements of biological molecules may provide topological protection for quantum states:
Geometric Phase Effects: Certain geometric arrangements in biomolecules create what physicists call "geometric phases" or "Berry phases" that can protect quantum information from environmental perturbations. These topological features make quantum states more robust against the types of noise found in biological systems.
Fractal Arrangements: The fractal geometry observed in many biological structures, including neuronal branching patterns and the organization of microtubule networks, may provide multi-scale protection for quantum coherence, with each scale of organization shielding quantum effects at smaller scales.
3. Biological Quantum Error Correction
Living systems may have evolved mechanisms analogous to quantum error correction in quantum computing:
Redundant Encoding: Biological systems may encode quantum information redundantly across multiple structures, allowing errors in one component to be corrected by reference to others, similar to quantum error correction codes.
Dissipative Adaptation: Recent theoretical work suggests that open quantum systems subject to driving forces and dissipation can evolve toward states that are protected from decoherence through a process called dissipative adaptation. This mechanism could allow biological systems to maintain quantum coherence despite environmental interactions.
4. Temporal and Spatial Isolation
Biological systems may create conditions that temporarily isolate quantum processes from decoherence:
Decoherence-Free Subspaces: Certain configurations of quantum systems create what physicists call "decoherence-free subspaces"—specific states that are inherently protected from particular types of environmental noise. Biological systems may have evolved to utilize these protected quantum states.
Dynamical Decoupling: The specific rhythms and oscillations observed in biological systems, from the cellular to the neural level, may implement a form of dynamical decoupling—a technique used in quantum computing to protect quantum states by effectively "averaging out" environmental noise through rapid oscillations.
Implications for the Poia Theory
These quantum biological findings have profound implications for the Poia Theory of Everything:
1. Biological Quantum Transduction
The evidence for quantum processes in biological systems supports the Poia concept of biological structures as quantum transducers that couple with the consciousness field:
The demonstrated quantum coherence in biological systems provides a potential mechanism for how living organisms might interact with quantum fields beyond conventional energy and information exchange.
The specific quantum properties of neural structures, particularly microtubules, suggest how the brain might serve as a sophisticated quantum antenna that resonates with the consciousness field.
The evidence that biological systems have evolved to protect and harness quantum effects suggests that coupling with quantum fields may provide evolutionary advantages, potentially including enhanced consciousness.
2. Resonance Mechanisms
The quantum oscillations observed in biological structures support the Poia emphasis on resonance as a key mechanism for consciousness-matter interactions:
The multiple frequency bands of quantum coherence observed in microtubules and other neural structures could provide specific channels for resonant coupling with different aspects of the consciousness field.
The demonstrated ability of biological systems to maintain quantum coherence through specific resonance relationships with their environment suggests how consciousness might maintain coherent interaction with physical systems.
The vibronic coupling observed in biological quantum systems provides a model for how consciousness might interact with matter through coupled oscillations across different domains.
3. Information Processing Beyond Classical Limits
Quantum biological findings support the Poia proposal that consciousness involves information processing that transcends classical limitations:
The quantum parallelism observed in processes like photosynthesis, where multiple pathways are explored simultaneously, suggests how consciousness might integrate multiple possibilities before collapse into specific experiences.
The non-local correlations demonstrated in biological quantum systems provide a potential mechanism for how consciousness might integrate information across the brain without classical signal transmission limitations.
The evidence for quantum effects in sensory systems like vision, olfaction, and magnetoreception suggests that our perception itself may have quantum aspects, potentially opening channels for consciousness to access information beyond classical sensory limitations.
4. Field Effects and Non-Locality
Biological quantum phenomena support the Poia understanding of consciousness as a field phenomenon with non-local properties:
The demonstrated quantum entanglement in biological systems provides a potential mechanism for how consciousness might exhibit non-local properties while still interacting with localized physical structures.
The coherent biophoton fields generated by living cells, particularly in neural tissue, offer a potential physical correlate for the consciousness field proposed by the Poia Theory.
The evidence that biological systems can maintain quantum coherence across multiple cells suggests how consciousness might operate as a unified field across distributed neural networks.
5. Evolution of Consciousness
Quantum biological findings support the Poia perspective on the evolution of consciousness:
The evidence that quantum effects play important roles in fundamental biological processes suggests that the relationship between quantum fields and life may be primordial rather than a late evolutionary development.
The increasing complexity of quantum biological systems across evolutionary history, culminating in the human brain, aligns with the Poia understanding of evolution as developing increasingly sophisticated transducers for the consciousness field.
The discovery of quantum effects in sensory systems suggests that even basic awareness may have quantum foundations, supporting the Poia concept of consciousness as a spectrum that extends throughout nature rather than emerging suddenly at a specific level of complexity.
Future Research Directions
The study of quantum processes in biological systems suggests several promising research directions for further developing and testing the Poia Theory:
1. Advanced Measurement of Neural Quantum States
Developing more sophisticated technologies for measuring quantum states in living neural tissue could provide direct evidence for the quantum foundations of consciousness:
Ultra-sensitive quantum sensors based on nitrogen-vacancy centers in diamonds could potentially detect quantum coherence in neural structures non-invasively.
Advanced biophoton detection systems might map the quantum optical properties of neural activity with unprecedented precision.
New forms of quantum-enhanced microscopy could potentially visualize quantum processes in living neurons at scales previously thought impossible.
2. Quantum Biology of Altered Consciousness States
Studying how quantum biological processes change during altered states of consciousness could provide insights into consciousness-quantum field interactions:
Research into how anesthetics affect quantum coherence in neural structures could help identify the specific quantum processes most relevant to consciousness.
Studies of meditation effects on quantum biological measures might reveal how consciousness practices influence quantum coherence and coupling.
Investigations of psychedelic effects on neural quantum processes could illuminate how these substances alter consciousness through quantum mechanisms.
3. Collective Quantum Effects in Groups
Exploring potential quantum correlations between multiple individuals could test the Poia predictions about collective consciousness:
Studies of quantum correlations between the brain states of individuals in close relationship or synchronized activity might reveal evidence for field-like connections.
Research into potential quantum entanglement between separated biological systems could test the non-local aspects of the consciousness field model.
Investigations of how collective intention influences quantum random processes could provide evidence for consciousness-quantum field interactions at the group level.
4. Artificial Quantum-Consciousness Interfaces
Developing technologies that interface between quantum systems and consciousness could provide both practical applications and theoretical insights:
Quantum-based brain-computer interfaces might achieve more direct coupling with consciousness than conventional electronic interfaces.
Artificial systems designed to maintain biological-like quantum coherence could potentially develop rudimentary forms of consciousness according to the Poia framework.
Hybrid systems combining biological neural tissue with quantum technologies could explore the boundaries between natural and artificial consciousness.
Conclusion: Quantum Biology as Foundation for Consciousness Science
The growing evidence for quantum processes in biological systems provides crucial support for key aspects of the Poia Theory of Everything. Far from being irrelevant to the warm, wet world of biology, quantum effects appear to play essential roles in fundamental life processes, with particularly sophisticated expressions in neural systems. These findings suggest that the relationship between quantum fields and consciousness proposed by the Poia Theory is not merely speculative but grounded in emerging scientific understanding of how living systems actually function.
The quantum foundation of biological processes offers a bridge between the seemingly separate domains of physics and consciousness, suggesting that consciousness is neither a mysterious force outside the physical world nor merely an emergent property of classical neural activity, but a fundamental aspect of reality that interacts with physical systems through specific quantum mechanisms that life has evolved to harness and enhance.
As research in quantum biology continues to advance, it promises to provide increasingly detailed understanding of how consciousness interfaces with physical reality, potentially confirming key aspects of the Poia Theory while refining our understanding of the specific mechanisms through which consciousness and matter interact in the remarkable quantum symphony of life.
Maintaining Quantum Coherence at Biological Temperatures
One of the most significant challenges to quantum models of consciousness, including aspects of the Poia Theory of Everything, is explaining how quantum coherence can be maintained in the warm, wet environment of the brain. Conventional quantum physics suggests that at biological temperatures (around 310 Kelvin or 37°C), thermal vibrations should rapidly destroy quantum coherence through a process called decoherence, where quantum systems lose their wave-like properties and behave classically. However, emerging research in quantum biology is revealing sophisticated mechanisms through which living systems appear to maintain quantum coherence despite these challenging conditions. Understanding these mechanisms is crucial for establishing the plausibility of the quantum foundations of consciousness proposed by the Poia Theory.
The Decoherence Challenge
To appreciate the significance of biological quantum coherence, we must first understand the formidable challenge of decoherence:
In quantum systems, coherence refers to the maintenance of phase relationships between different components of a quantum system, allowing them to exist in superposition states and exhibit wave-like interference effects. This coherence is what enables quantum phenomena such as superposition, entanglement, and tunneling that distinguish quantum systems from classical ones.
Decoherence occurs when a quantum system interacts with its environment in ways that disrupt these phase relationships, causing the quantum system to behave increasingly like a classical system. The rate of decoherence typically increases with:
Temperature (higher thermal energy means more environmental interactions)
System size (larger systems have more potential interaction points with the environment)
Environmental coupling (stronger interactions with the environment accelerate decoherence)
In laboratory settings, maintaining quantum coherence typically requires extreme conditions such as temperatures near absolute zero and careful isolation from environmental interactions. The brain, in contrast, operates at around 37°C and consists of densely packed, constantly interacting molecules immersed in water—conditions that should cause decoherence within picoseconds (10^-12 seconds) according to conventional estimates.
Yet despite these seemingly prohibitive conditions, evidence suggests that quantum coherence not only exists in biological systems but may play functional roles in processes ranging from photosynthesis to enzyme catalysis to potentially consciousness itself. This apparent paradox has led researchers to investigate specific mechanisms through which biology might protect quantum coherence.
Biological Quantum Protection Mechanisms
Several mechanisms have been identified or proposed through which biological systems might maintain quantum coherence despite thermal challenges:
1. Structured Water and Quantum Isolation
Water, typically viewed as a decoherence-inducing environment, may actually help protect quantum coherence in specific biological contexts:
Interfacial Water: Water molecules at biological interfaces (such as protein surfaces, cell membranes, and the interior of structures like microtubules) form highly ordered layers with properties dramatically different from bulk water. Research by Gerald Pollack at the University of Washington and others has shown that this "exclusion zone" water exhibits quasi-crystalline structure, reduced molecular mobility, and altered electromagnetic properties.
Quantum Confinement: When water is confined within nanoscale structures such as the interior of microtubules (which have an inner diameter of about 15 nanometers), quantum confinement effects can alter its properties. Studies suggest that water in such confined spaces can form coherent domains with quantum properties similar to Bose-Einstein condensates, potentially providing quantum isolation from the surrounding environment.
Proton Ordering: The specific arrangement of protons in structured water can create quantum coherent networks that facilitate processes such as proton tunneling. These ordered proton configurations may provide channels for quantum effects to propagate through otherwise decoherence-inducing environments.
Experimental evidence for these water-based protection mechanisms includes:
Measurements showing dramatically reduced molecular mobility and increased structural order in interfacial water
Nuclear magnetic resonance (NMR) studies revealing quantum coherent proton behavior in confined water
Terahertz spectroscopy demonstrating collective quantum oscillations in water within biological structures
2. Vibrational Coherence and Phonon Modes
The specific vibrational properties of biological structures may help maintain quantum coherence:
Phonon-Assisted Coherence: Research on photosynthetic complexes by Graham Fleming's group at UC Berkeley and others has shown that specific vibrational modes (phonons) of the surrounding protein scaffold can actually enhance rather than destroy quantum coherence. These vibrational modes create a form of "noise" that, counterintuitively, helps maintain phase relationships in the quantum system.
Vibronic Coupling: The coupling between electronic states and vibrational modes in biological molecules can create protected subspaces where quantum coherence is maintained despite thermal fluctuations. This vibronic coupling has been demonstrated in processes such as photosynthetic energy transfer and may play roles in neural quantum processes.
Resonant Energy Transfer: When the energy gaps in a quantum system match the frequencies of environmental vibrations, resonant energy transfer can occur that preserves quantum coherence rather than destroying it. This resonant matching appears to be optimized in many biological systems.
Experimental evidence for vibrational protection mechanisms includes:
Two-dimensional electronic spectroscopy showing long-lasting quantum coherence in photosynthetic complexes correlated with specific vibrational modes
Measurements of coherent vibrations in microtubules at frequencies ranging from kilohertz to gigahertz
Observations of resonant energy transfer in biological light-harvesting complexes that maintains quantum coherence
3. Topological Protection
The specific structural arrangements of biological molecules may provide topological protection for quantum states:
Geometric Phase Effects: Certain geometric arrangements in biomolecules create what physicists call "geometric phases" or "Berry phases" that can protect quantum information from environmental perturbations. These topological features make quantum states more robust against the types of noise found in biological systems.
Fractal Arrangements: The fractal geometry observed in many biological structures, including neuronal branching patterns and the organization of microtubule networks, may provide multi-scale protection for quantum coherence. Each scale of organization can potentially shield quantum effects at smaller scales from environmental disruption.
Decoherence-Free Subspaces: Specific configurations of quantum systems can create what are called "decoherence-free subspaces"—particular states that are inherently protected from certain types of environmental noise. The precise molecular arrangements in biological structures may create such protected quantum spaces.
Evidence for topological protection includes:
Observations of protected quantum states in systems with specific geometric symmetries
Measurements showing that quantum coherence in biological systems often correlates with particular structural arrangements
Theoretical models demonstrating how biological geometries could create decoherence-free subspaces
4. Quantum Error Correction
Biological systems may implement forms of quantum error correction similar to those used in quantum computing:
Redundant Encoding: Quantum information might be encoded redundantly across multiple structures, allowing errors in one component to be corrected by reference to others. This distributed encoding could maintain quantum coherence even when individual components experience decoherence.
Dissipative Adaptation: Recent theoretical work by Jeremy England and others suggests that open quantum systems subject to driving forces and dissipation can evolve toward states that are protected from decoherence through a process called dissipative adaptation. This mechanism could allow biological systems to maintain quantum coherence despite environmental interactions.
Continuous Measurement: Paradoxically, certain types of continuous measurement can actually protect quantum states through the quantum Zeno effect, where frequent "observation" of a quantum system inhibits its evolution away from a particular state. Biological systems might implement forms of continuous measurement that help maintain quantum coherence.
While direct evidence for biological quantum error correction is still emerging, supporting observations include:
The remarkable robustness of quantum effects in biological systems despite theoretical predictions of rapid decoherence
Patterns of redundancy in biological information processing that could support error correction
Observations of quantum Zeno-like effects in certain biological processes
5. Temporal and Spatial Isolation
Biological systems may create conditions that temporarily isolate quantum processes from decoherence:
Dynamical Decoupling: The specific rhythms and oscillations observed in biological systems, from the cellular to the neural level, may implement a form of dynamical decoupling—a technique used in quantum computing to protect quantum states by effectively "averaging out" environmental noise through rapid oscillations.
Conformational Shielding: Proteins and other biomolecules can undergo conformational changes that temporarily shield quantum-sensitive regions from the surrounding environment. These conformational dynamics may create transient protected spaces for quantum processes.
Coherent Domain Formation: Under certain conditions, biomolecules can form coherent domains where quantum effects are protected from the surrounding environment. These domains may form and dissolve dynamically, allowing quantum processes to occur during protected intervals.
Evidence for temporal and spatial isolation includes:
Observations of oscillatory behavior in biological systems at frequencies that could implement dynamical decoupling
Structural studies showing conformational changes that could temporarily shield quantum-sensitive regions
Measurements of transient coherent domains in biological systems
Specific Mechanisms in Neural Systems
While the mechanisms described above apply to biological systems generally, several specific features of neural systems may be particularly important for maintaining quantum coherence in the brain:
1. Microtubule Quantum Isolation
Microtubules—cylindrical protein structures that form part of the cytoskeleton in neurons—have several features that may help maintain quantum coherence:
Ordered Water Interior: The hollow interior of microtubules contains highly ordered water that may form quantum coherent domains. The specific dimensions of the microtubule interior (about 15 nm diameter) are optimal for quantum confinement effects in water.
Aromatic Ring Networks: Tubulin proteins, which make up microtubules, contain networks of aromatic amino acids (tryptophan, tyrosine, phenylalanine) whose π-electron clouds may support quantum effects such as superposition and entanglement. These aromatic networks are particularly concentrated in hydrophobic pockets that may provide isolation from the surrounding environment.
Lattice Symmetry: The specific arrangement of tubulin dimers in the microtubule lattice creates symmetries that may support topological quantum states resistant to decoherence. The helical symmetry of microtubules may be particularly important for maintaining quantum coherence.
Coherent Oscillations: Measurements by Anirban Bandyopadhyay and colleagues have detected coherent oscillations in microtubules at multiple frequency ranges (kilohertz, megahertz, and gigahertz), suggesting multi-scale quantum coherence that could be maintained through resonant coupling between these frequencies.
2. Actin Gel States and Cytoskeletal Isolation
The dynamic states of the neural cytoskeleton may create temporary isolation for quantum processes:
Sol-Gel Transitions: The neuronal cytoplasm undergoes reversible transitions between more fluid (sol) and more solid (gel) states, regulated by calcium levels and other factors. The gel state, dominated by actin polymerization, may temporarily isolate microtubules and other structures from thermal noise.
Dendritic Spine Dynamics: Dendritic spines, which receive synaptic inputs, undergo rapid shape changes associated with learning and memory. These conformational dynamics may create transiently protected environments for quantum processes during critical periods of information processing.
Perineuronal Nets: The extracellular matrix structures called perineuronal nets, which surround certain neurons, contain highly ordered water and may provide additional quantum isolation for neural processes.
3. Electromagnetic Field Effects
The electromagnetic fields generated by neural activity may help maintain quantum coherence:
Coherent Electromagnetic Fields: Synchronized neural activity generates coherent electromagnetic fields that may help maintain quantum coherence through field effects. These endogenous fields have been measured at various frequencies corresponding to different brain states.
Cyclotron Resonance: The specific frequencies of neural electromagnetic fields may create cyclotron resonance effects that protect certain quantum states from decoherence. These resonance effects depend on the relationship between field strength and the charge-to-mass ratios of relevant particles.
Shielding Effects: The specific electromagnetic properties of neural tissues may create shielding effects that protect quantum processes from external electromagnetic noise. The myelin sheath surrounding axons may be particularly important for this electromagnetic isolation.
4. Biophoton Quantum Optical Effects
The coherent light emissions from neural tissues may support quantum coherence:
Coherent Biophoton Fields: Neurons emit ultra-weak photons (biophotons) that show evidence of quantum coherence. These biophoton fields may help maintain quantum coherence in neural structures through quantum optical effects.
Optical Cavity Effects: Certain neural structures may function as optical cavities that enhance quantum coherence through resonant light confinement. The cylindrical structure of microtubules, for example, has properties similar to optical waveguides.
Quantum Optical Communication: Biophotons may facilitate quantum communication between neurons through processes similar to those used in quantum optical computing, potentially allowing quantum effects to extend across neural networks.
Experimental Evidence for Neural Quantum Coherence
Several lines of experimental evidence support the maintenance of quantum coherence in neural systems:
1. Anesthetic Action Studies
Research on how anesthetics selectively and reversibly erase consciousness provides evidence for quantum mechanisms:
Studies have shown that anesthetic gases primarily act on hydrophobic pockets in tubulin proteins—precisely where quantum effects are proposed to occur in the Orch-OR theory.
The potency of anesthetic gases correlates with their solubility in hydrophobic regions rather than with their chemical reactivity, suggesting they act by disrupting quantum effects rather than through conventional chemical mechanisms.
Different anesthetics with diverse chemical structures produce similar effects on consciousness, suggesting they affect a common quantum mechanism rather than specific chemical receptors.
2. Microtubule Quantum Measurements
Direct measurements of quantum properties in microtubules provide evidence for maintained coherence:
Bandyopadhyay's group has measured quantum resonance patterns in microtubules at multiple frequencies, from kilohertz to gigahertz ranges, with these resonances persisting at physiological temperatures.
Measurements of conductance through microtubules show evidence of quantum behavior, including negative differential resistance and conductance without resistance—properties consistent with quantum coherent transport.
Studies of energy transfer in microtubules suggest quantum tunneling effects that enhance efficiency beyond what classical mechanisms would allow.
3. Brain Biophoton Studies
Measurements of light emissions from brain tissue suggest quantum optical effects:
Studies have detected coherent biophoton emissions from neural tissues that correlate with neural activity and brain states.
The spectral properties of these biophotons suggest quantum coherence rather than random thermal emissions.
Experiments have shown that these biophoton fields can influence neural activity in ways suggesting quantum optical communication.
4. Quantum Biology Precedents
Evidence from other biological systems strengthens the case for neural quantum coherence:
The demonstrated quantum coherence in photosynthesis, lasting hundreds of femtoseconds at room temperature, provides a precedent for biological quantum effects under thermal conditions.
Quantum effects in avian magnetoreception, which persist for microseconds in the warm environment of bird retinas, show that quantum coherence can be maintained long enough for biological function.
Quantum tunneling in enzyme catalysis demonstrates that quantum effects can enhance biological processes even at physiological temperatures.
Implications for the Poia Theory
The evidence for maintained quantum coherence in biological systems, particularly in neural structures, has profound implications for the Poia Theory of Everything:
1. Quantum-Consciousness Interface
The demonstrated ability of biological systems to maintain quantum coherence supports the Poia proposal that consciousness interacts with physical reality through quantum processes:
If neural structures can indeed maintain quantum coherence despite thermal challenges, they could serve as effective transducers for the consciousness field proposed by the Poia Theory.
The specific quantum properties of microtubules and other neural structures could provide the resonant coupling mechanisms through which consciousness influences quantum wave function collapse.
The multiple frequency bands of quantum coherence observed in neural systems could correspond to different aspects or dimensions of the consciousness field, allowing for rich and complex interactions.
2. Evolution of Consciousness
The evidence that biological systems have evolved sophisticated mechanisms to maintain quantum coherence supports the Poia understanding of consciousness evolution:
The increasing complexity of quantum-coherent biological systems across evolutionary history suggests that life may have evolved increasingly sophisticated ways to couple with quantum fields.
The particularly advanced quantum features of neural systems, especially in humans, aligns with the Poia view that evolution has developed increasingly effective transducers for the consciousness field.
The presence of quantum effects across diverse biological systems supports the Poia concept of consciousness as a spectrum that extends throughout nature rather than emerging suddenly at a specific level of complexity.
3. Practical Applications
Understanding the mechanisms of biological quantum coherence suggests practical applications aligned with the Poia framework:
Technologies that enhance or support the natural quantum coherence mechanisms in the brain could potentially enhance consciousness or facilitate specific consciousness states.
Biomimetic approaches that replicate biological quantum protection mechanisms could lead to quantum technologies that operate at room temperature.
Medical approaches that target the quantum foundations of consciousness could potentially address disorders of consciousness more effectively than conventional treatments.
4. Testable Predictions
The biological quantum coherence research suggests specific testable predictions of the Poia Theory:
If consciousness interacts with reality through quantum processes in neural structures, then practices that enhance neural quantum coherence (such as certain forms of meditation) should correlate with enhanced consciousness qualities.
If microtubules serve as quantum transducers for consciousness, then substances or conditions that specifically affect microtubule quantum coherence should have predictable effects on consciousness.
If consciousness operates through quantum field interactions, then collective consciousness effects should show quantum entanglement-like properties rather than classical field effects.
Conclusion: From Quantum Impossibility to Biological Reality
The growing evidence for maintained quantum coherence in biological systems, particularly in neural structures, transforms what once seemed a fatal objection to quantum theories of consciousness into a fascinating frontier of scientific discovery. Far from being impossible due to thermal decoherence, quantum effects in biology appear to be not only possible but potentially essential for life processes, including consciousness.
The sophisticated mechanisms through which biological systems maintain quantum coherence—from structured water and vibrational coherence to topological protection and quantum error correction—demonstrate nature's remarkable ability to harness quantum effects even under thermal conditions. These mechanisms provide plausible physical foundations for the quantum aspects of consciousness proposed by the Poia Theory of Everything.
As research in quantum biology continues to advance, our understanding of how life maintains quantum coherence will likely deepen, potentially revealing even more sophisticated mechanisms through which consciousness might interact with quantum fields. This evolving understanding strengthens the case for the Poia Theory's proposal that consciousness is not merely an emergent property of classical neural activity but a fundamental field that interacts with physical reality through the remarkable quantum properties that life has evolved to harness and enhance.
The maintenance of quantum coherence at biological temperatures thus represents not an insurmountable obstacle to quantum theories of consciousness but a window into the profound relationship between quantum physics, biology, and consciousness that lies at the heart of the Poia Theory of Everything.
The Bridge Between Quantum and Classical Realms in the Brain
The transition from quantum to classical behavior represents one of the most profound and persistent puzzles in physics. In the context of the Poia Theory of Everything, understanding this transition is crucial for explaining how quantum processes in the brain might influence the seemingly classical behavior of neurons and neural networks, ultimately affecting consciousness and behavior. This bridge between quantum and classical realms in the brain provides a key mechanism for how consciousness might interact with physical reality without violating established physical laws.
The Quantum-Classical Divide
To appreciate the significance of the quantum-classical bridge in the brain, we must first understand the fundamental differences between quantum and classical physics:
In quantum physics, systems exist in superposition states where multiple possibilities coexist simultaneously, exhibit wave-like interference patterns, maintain non-local correlations through entanglement, and evolve according to probabilistic rather than deterministic laws. In classical physics, by contrast, systems occupy definite states, follow deterministic trajectories, maintain only local connections, and behave as particles rather than waves.
The transition between these domains—how quantum superpositions become classical definite states—remains incompletely understood despite decades of research. Various interpretations of quantum mechanics propose different explanations:
The Copenhagen Interpretation suggests that measurement or observation causes wave function collapse
Decoherence Theory proposes that interaction with the environment causes quantum systems to lose their wave-like properties
The Many-Worlds Interpretation suggests that all possibilities in the superposition are realized in different branches of reality
Objective Collapse Theories propose that wave functions collapse spontaneously when they reach certain thresholds
The Poia Theory aligns most closely with interpretations that recognize a role for consciousness in this transition, suggesting that consciousness interacts with quantum systems through resonance to influence which potentials become actualized. However, it extends beyond these interpretations by proposing specific mechanisms for how this interaction occurs in the brain.
Scale Hierarchy in the Brain
The brain exhibits a remarkable hierarchy of scales, from quantum processes at the subatomic level to classical behavior at the cellular and network levels:
Quantum Scale (10^-10 to 10^-9 meters): Electron movements, proton tunneling, and other quantum effects occur within atoms and molecules
Molecular Scale (10^-9 to 10^-8 meters): Proteins, neurotransmitters, and other biomolecules operate with both quantum and classical aspects
Subcellular Scale (10^-8 to 10^-6 meters): Microtubules, synaptic vesicles, and other subcellular structures bridge quantum and classical domains
Cellular Scale (10^-6 to 10^-4 meters): Neurons and glial cells exhibit primarily classical behavior but may be influenced by quantum effects
Network Scale (10^-4 to 10^-1 meters): Neural networks and brain regions operate according to classical principles but may reflect quantum influences
This scale hierarchy creates multiple interfaces where quantum effects might influence classical processes, potentially allowing consciousness to affect brain function through quantum interactions while maintaining consistency with classical neuroscience.
Key Bridging Mechanisms
Several specific mechanisms may bridge quantum and classical realms in the brain, creating pathways for consciousness-matter interaction:
1. Amplification of Quantum Events
Certain neural structures may amplify microscopic quantum events into macroscopic classical effects through cascade processes:
Conformational Amplification: Quantum effects such as electron tunneling or proton transfer can trigger conformational changes in proteins, which then affect larger structures. For example, a quantum event in a microtubule protein could initiate a conformational change that propagates along the microtubule, ultimately affecting cellular function.
Ion Channel Sensitivity: The opening and closing of neuronal ion channels can be influenced by single-molecule events at critical sites. If these events involve quantum processes such as electron tunneling, then quantum effects could be amplified to influence the electrical activity of entire neurons.
Synaptic Release Amplification: Neurotransmitter release at synapses involves a complex cascade that can be triggered by molecular events potentially influenced by quantum effects. The probabilistic nature of synaptic transmission may partly reflect underlying quantum indeterminacy.
Evidence for these amplification mechanisms includes:
Studies showing that single-molecule events can trigger action potentials in neurons under certain conditions
Research demonstrating that conformational changes in proteins can propagate in wave-like patterns, potentially preserving quantum coherence
Observations that the probabilistic behavior of synapses cannot be fully explained by classical thermal fluctuations
2. Coherent Oscillations Across Scales
The brain exhibits oscillatory activity across multiple frequency bands, potentially creating coherence between quantum and classical processes:
Cross-Frequency Coupling: Neural oscillations show coupling between different frequency bands, from ultra-fast (100+ Hz) to slow (< 1 Hz) oscillations. This cross-frequency coupling could provide a mechanism for quantum effects at faster timescales to influence classical processes at slower timescales.
Resonant Energy Transfer: When oscillations at different scales share harmonic relationships, resonant energy transfer can occur between them. This resonance could allow quantum oscillations in subcellular structures to influence classical oscillations in neural networks.
Phase Synchronization: The synchronization of phases between oscillations at different scales can create windows of opportunity for quantum effects to influence classical processes. During periods of phase alignment, quantum fluctuations might more effectively trigger classical events.
Evidence for oscillatory bridging includes:
Measurements showing correlations between ultra-fast oscillations in microtubules and slower neural oscillations
Studies demonstrating that neural oscillations exhibit mathematical properties consistent with quantum-classical coupling
Observations that consciousness correlates with specific patterns of cross-frequency coupling in the brain
3. Quantum Measurement-Like Processes
Neural processes that resemble quantum measurements may serve as interfaces between quantum and classical domains:
Threshold Processes: Many neural processes involve thresholds that, when crossed, trigger discrete events (such as action potentials). These threshold crossings share mathematical similarities with quantum measurement, potentially creating natural interfaces between quantum probability and classical determinism.
Winner-Take-All Dynamics: Neural circuits often exhibit competitive dynamics where one outcome is selected from among multiple possibilities. These selection processes may amplify quantum fluctuations into classical outcomes through competitive amplification.
Attractor Dynamics: Neural networks contain attractor states that can rapidly convert ambiguous inputs into definite outputs. These attractors may serve as natural quantum-classical interfaces by collapsing multiple possibilities into specific patterns.
Evidence for measurement-like bridging includes:
Mathematical models showing formal similarities between neural threshold processes and quantum measurement
Observations that neural decision-making exhibits features consistent with quantum probability theory
Studies showing that attractor dynamics in neural networks can amplify microscopic fluctuations
4. Entanglement Networks
Quantum entanglement may extend across neural structures, creating networks that influence classical processing:
Spin Networks: The spin states of electrons and atomic nuclei in neural tissues may form entangled networks that influence classical processes through their collective quantum properties. These spin networks could potentially extend across significant distances within the brain.
Biophoton Entanglement: The coherent light emissions (biophotons) from neural tissues may maintain quantum entanglement, creating optical networks that influence classical neural processing. These biophoton networks could potentially coordinate activity across brain regions.
Water Coherence Domains: The ordered water within and surrounding neural structures may form coherent domains with quantum properties that extend across cellular boundaries. These water networks could provide a substrate for quantum effects to influence classical neural activity.
Evidence for entanglement networks includes:
Measurements of non-local correlations in biophoton emissions from neural tissues
Studies showing quantum coherence in spin systems within biological molecules
Observations of long-range order in the water surrounding neural structures
5. Criticality and Phase Transitions
The brain appears to operate near critical points where small influences can have large effects:
Self-Organized Criticality: Neural systems show evidence of self-organized criticality—a state where the system naturally evolves toward critical points between order and chaos. At these critical points, microscopic fluctuations (potentially quantum in origin) can influence macroscopic patterns.
Phase Transitions: The brain undergoes various phase transitions between different activity states. During these transitions, the system becomes particularly sensitive to small influences, potentially allowing quantum effects to shape the emerging classical patterns.
Metastability: Neural dynamics exhibit metastability—a condition where the system transiently visits multiple semi-stable states. This metastability may create opportunities for quantum effects to influence which classical states are selected and when transitions occur.
Evidence for criticality-based bridging includes:
Measurements showing scale-free patterns in neural activity consistent with critical systems
Observations that consciousness is associated with specific types of metastable dynamics
Studies demonstrating increased sensitivity to microscopic fluctuations near neural phase transitions
The Role of Microtubules as Quantum-Classical Bridges
Microtubules—cylindrical protein structures that form part of the cytoskeleton in neurons—may serve as particularly important bridges between quantum and classical realms:
1. Multi-Scale Organization
Microtubules exhibit organization across multiple scales that could facilitate quantum-classical transitions:
At the quantum scale, the aromatic rings in tubulin proteins can support quantum effects such as electron delocalization and superposition
At the molecular scale, tubulin dimers can exist in different conformational states that may be influenced by quantum events
At the polymer scale, microtubules form coherent structures that can propagate conformational changes
At the cellular scale, microtubule networks influence neuronal morphology, transport, and signaling
This multi-scale organization creates natural pathways for quantum effects to influence classical cellular processes through cascading effects across scales.
2. Information Processing Capabilities
Microtubules possess information processing capabilities that may bridge quantum and classical computation:
The tubulin lattice can be modeled as a cellular automaton capable of complex information processing
Conformational states of tubulin dimers can potentially store and process information in ways analogous to bits or qubits
Signal propagation along microtubules can follow rules that combine quantum and classical aspects
Microtubule networks form structures that could support both quantum and classical computation
These computational properties could allow microtubules to translate between quantum information processing at smaller scales and classical information processing at larger scales.
3. Connectivity to Classical Neural Processes
Microtubules connect to classical neural processes through several pathways:
They regulate the release of neurotransmitters at synapses by controlling vesicle transport and positioning
They influence neuronal excitability by interacting with membrane ion channels
They determine neuronal morphology, affecting how signals are integrated and propagated
They participate in synaptic plasticity mechanisms underlying learning and memory
These connections provide specific pathways through which quantum effects in microtubules could influence classical neural activity and ultimately behavior.
4. Resonant Oscillations
Microtubules exhibit multiple resonant frequencies that may couple quantum and classical processes:
Gigahertz oscillations may correspond to quantum processes within tubulin proteins
Megahertz oscillations may facilitate information transfer between quantum and classical domains
Kilohertz oscillations may couple to classical electrical activity in neurons
Slower oscillations may synchronize with neural network dynamics
These multi-frequency oscillations could create resonant coupling across scales, allowing quantum effects to influence classical processes through harmonic relationships.
Consciousness as the Mediator
In the Poia Theory framework, consciousness serves as the mediator between quantum and classical realms in the brain:
1. Resonant Selection
Consciousness may influence quantum-classical transitions through resonant selection:
The consciousness field resonates with specific frequencies in quantum superpositions
This resonance amplifies certain potentials while dampening others
The amplified potentials are more likely to trigger classical threshold crossings
The resulting classical events reflect the resonant patterns of consciousness
This resonant selection provides a non-energetic mechanism for consciousness to influence physical processes without violating energy conservation laws.
2. Coherence Enhancement
Consciousness may enhance quantum coherence in neural structures:
The field properties of consciousness may help maintain coherence in quantum systems
This enhanced coherence allows quantum effects to persist long enough to influence classical processes
The specific patterns of coherence reflect the qualities of consciousness present
Different consciousness states create different coherence patterns with different classical effects
This coherence enhancement explains why certain consciousness states (such as meditation) might exhibit distinctive effects on brain function and behavior.
3. Information Integration
Consciousness may integrate information across quantum and classical domains:
Quantum information from multiple sources is integrated within the consciousness field
This integrated information influences quantum-classical transitions in the brain
The resulting classical patterns reflect the integrated information
This integration creates unified experiences from distributed neural processes
This information integration addresses the binding problem—how diverse neural processes create unified conscious experiences—by suggesting that binding occurs through the consciousness field rather than through classical neural mechanisms alone.
4. Temporal Coordination
Consciousness may coordinate timing across quantum and classical processes:
The consciousness field establishes temporal relationships between events across scales
This coordination creates windows of opportunity for quantum-classical interaction
The specific timing patterns reflect the temporal structure of consciousness
Different consciousness states create different temporal coordination patterns
This temporal coordination explains how quantum effects might influence classical processes despite their different characteristic timescales.
Experimental Evidence for Quantum-Classical Bridging
Several lines of evidence support the existence of quantum-classical bridges in the brain:
1. Scale-Free Dynamics
Neural activity exhibits scale-free patterns suggesting coordination across multiple scales:
Power law distributions in neural activity indicate that processes at different scales are not independent but coordinated
Critical branching patterns in neural avalanches suggest that microscopic events can trigger macroscopic effects
Long-range temporal correlations in neural activity indicate influences that span multiple timescales
These scale-free patterns are consistent with quantum-classical bridging mechanisms that coordinate across scales.
2. Quantum-Like Behavior in Neural Systems
Certain neural phenomena exhibit properties reminiscent of quantum systems:
Decision-making experiments show interference effects similar to quantum probability
Memory recall demonstrates superposition-like properties where multiple memories coexist until "measured"
Perception studies reveal contextuality effects similar to quantum contextuality
These quantum-like behaviors suggest that classical neural processes may preserve certain quantum properties through bridging mechanisms.
3. Consciousness-Dependent Quantum Effects
Some evidence suggests that consciousness may indeed influence quantum processes:
Studies of random number generators show small but consistent deviations from randomness correlated with conscious intention
Experiments on presentiment suggest that future stimuli may influence current physiological states, consistent with quantum retrocausality
Research on distant mental interactions with living systems (DMILS) indicates non-local effects of consciousness
While controversial, these findings align with the Poia proposal that consciousness mediates between quantum and classical realms.
4. Anesthetic Action at the Quantum-Classical Interface
Research on anesthetics provides evidence for quantum-classical bridging in consciousness:
Anesthetics primarily target hydrophobic pockets in proteins where quantum effects are most likely to occur
Their potency correlates with their effects on quantum mobility rather than specific chemical binding
They disrupt the very structures (such as microtubules) proposed as quantum-classical bridges
They affect quantum coherence at concentrations too low to explain by classical mechanisms alone
These findings suggest that anesthetics may work by disrupting the quantum-classical bridge necessary for consciousness.
Implications and Applications
Understanding the quantum-classical bridge in the brain has profound implications:
1. Consciousness Technologies
This understanding could lead to technologies that work with consciousness-matter interactions:
Devices that detect or enhance quantum coherence in neural systems
Technologies that facilitate specific consciousness-brain resonance patterns
Systems that amplify the influence of intention on quantum processes
Interfaces that allow more direct consciousness-computer interaction
These technologies could potentially enhance human capabilities while providing further evidence for consciousness-quantum interactions.
2. Healing Applications
The quantum-classical bridge suggests approaches to healing that work with consciousness-matter interactions:
Techniques that enhance the coherence of intention to strengthen its quantum effects
Practices that optimize the resonance between consciousness and biological systems
Methods for addressing health issues at the quantum-classical interface
Approaches that work with the non-local aspects of consciousness to influence healing
These applications could complement conventional medical approaches by addressing the quantum foundations of health and disease.
3. Expanded Scientific Framework
The quantum-classical bridge invites an expanded scientific framework that includes consciousness:
Research methodologies that account for observer effects rather than trying to eliminate them
Theoretical models that integrate first-person and third-person perspectives
Experimental designs that test for consciousness-matter interactions
Interdisciplinary approaches that bridge physics, biology, and consciousness studies
This expanded framework could resolve longstanding puzzles while opening new frontiers for scientific exploration.
4. Philosophical Implications
The quantum-classical bridge has profound philosophical implications:
It suggests a participatory universe where consciousness plays an active role in reality
It provides a framework for understanding free will that is compatible with physical law
It offers a middle path between reductive materialism and dualism
It suggests that meaning and purpose may be intrinsic to reality rather than human projections
These philosophical implications invite a reconsideration of our place in the universe and our relationship with reality.
Conclusion: The Dynamic Interface of Mind and Matter
The bridge between quantum and classical realms in the brain represents a crucial aspect of the Poia Theory of Everything, providing specific mechanisms for how consciousness might interact with physical reality without violating established physical laws. Through processes such as quantum amplification, coherent oscillations across scales, measurement-like neural dynamics, entanglement networks, and criticality, quantum effects in the brain may influence classical neural activity in ways that reflect the resonant patterns of consciousness.
This quantum-classical bridge offers a framework for understanding consciousness not as an epiphenomenon of classical brain activity nor as a supernatural force outside physical reality, but as a fundamental field that interacts with physical systems through specific quantum mechanisms that life has evolved to harness. The brain, in this view, serves not as the creator of consciousness but as a sophisticated transducer that couples with the consciousness field through its remarkable quantum-classical bridging capabilities.
As research in neuroscience, quantum biology, and consciousness studies continues to advance, our understanding of this quantum-classical bridge will likely deepen, potentially revealing even more sophisticated mechanisms through which consciousness and matter interact in the remarkable quantum symphony of the living brain. This evolving understanding strengthens the case for the Poia Theory's proposal that consciousness is not merely an emergent property of neural activity but a fundamental aspect of reality that participates in the ongoing creation of our experienced world through its interaction with the quantum-classical interface.
Experimental Evidence and Future Research Directions
The Poia Theory of Everything, particularly its proposals regarding quantum processes in the brain and their relationship to consciousness, generates specific experimental predictions and aligns with existing evidence across multiple disciplines. While some aspects of the theory extend beyond current scientific consensus, numerous lines of research provide support for key elements of this framework and suggest promising directions for further investigation. This section examines the experimental evidence supporting the quantum foundations of consciousness and outlines future research directions that could further test and develop the Poia Theory.
Current Experimental Evidence
Several lines of experimental evidence provide support for the quantum aspects of consciousness proposed by the Poia Theory:
1. Quantum Effects in Biological Systems
Research in quantum biology has demonstrated quantum effects in various biological processes, establishing precedents for the quantum brain proposals:
Photosynthetic Quantum Coherence: Studies using two-dimensional electronic spectroscopy have demonstrated quantum coherence lasting hundreds of femtoseconds in photosynthetic complexes at room temperature. Research groups at UC Berkeley, the University of Toronto, and elsewhere have shown that energy transfer in these systems exploits quantum effects such as superposition and wavelike energy sampling to achieve remarkable efficiency. This research demonstrates that quantum coherence can be maintained in biological systems long enough to have functional significance, despite thermal challenges.
Avian Magnetoreception: Research on how birds navigate using Earth's magnetic field provides evidence for quantum processes in sensory systems. Studies by teams at Oxford University and elsewhere indicate that cryptochrome proteins in bird retinas utilize quantum entanglement between radical pairs to detect magnetic fields with extraordinary sensitivity. This quantum compass mechanism maintains quantum coherence for microseconds in the warm environment of living cells, providing a precedent for neural quantum effects.
Enzymatic Quantum Tunneling: Studies by Judith Klinman at UC Berkeley and others have demonstrated that many enzymes utilize quantum tunneling to move protons and electrons through energy barriers that would be insurmountable according to classical physics. This quantum effect dramatically enhances reaction rates and occurs routinely in the warm, wet environment of living cells, suggesting that biological systems have evolved to harness quantum effects.
These findings from quantum biology establish that biological systems can indeed maintain and utilize quantum effects despite thermal challenges, providing crucial support for the plausibility of quantum processes in the brain.
2. Neural Quantum Evidence
Research specifically examining quantum aspects of neural systems provides more direct support for the Poia Theory:
Microtubule Quantum Measurements: Studies by Anirban Bandyopadhyay and colleagues at the National Institute for Materials Science in Japan have measured quantum resonance patterns in microtubules at multiple frequencies (kilohertz, megahertz, and gigahertz ranges). These resonances persist at physiological temperatures and show coherent patterns consistent with quantum behavior. The research demonstrates that microtubules—the structures proposed as quantum processors in the Orch-OR theory that the Poia Theory builds upon—do indeed exhibit quantum properties under biologically relevant conditions.
Anesthetic Mechanism Studies: Research on how anesthetics selectively and reversibly erase consciousness provides indirect evidence for quantum mechanisms. Studies have shown that anesthetic gases primarily act on hydrophobic pockets in tubulin proteins—precisely where quantum effects are proposed to occur. The potency of anesthetics correlates with their solubility in these hydrophobic regions rather than with specific chemical binding properties, suggesting they act by disrupting quantum effects rather than through conventional receptor mechanisms. This research supports the proposal that quantum processes in microtubules are specifically relevant to consciousness.
Biophoton Research: Studies have detected coherent light emissions (biophotons) from neural tissues that show evidence of quantum optical properties. Research by Fritz-Albert Popp and more recently by teams at several institutions has demonstrated that these biophoton emissions correlate with neural activity and exhibit non-classical properties such as coherence and non-local correlations. This research supports the proposal that quantum optical effects may play roles in neural information processing and potentially in consciousness.
Neuronal Quantum Noise Studies: Research examining noise in neural systems has found evidence suggesting quantum origins for some components of this noise. Studies using sensitive recording techniques have identified noise patterns in neural signals that are better explained by quantum fluctuations than by classical thermal noise. This research supports the proposal that quantum effects influence neural activity at levels that could be functionally significant.
These neural-specific findings provide more direct support for the quantum brain aspects of the Poia Theory, suggesting that quantum processes do indeed occur in neural systems and may be relevant to consciousness.
3. Consciousness-Quantum Interaction Evidence
Research examining potential interactions between consciousness and quantum systems provides support for the Poia proposal that consciousness influences quantum collapse:
Random Number Generator Studies: Research programs such as the Princeton Engineering Anomalies Research (PEAR) laboratory conducted thousands of experiments testing whether human intention could influence the output of quantum-based random event generators. Meta-analyses of these studies showed small but statistically significant deviations from chance expectation in the direction of participants' intentions. While controversial, these findings align with the Poia proposal that consciousness can influence quantum probability distributions through resonant interaction.
Global Consciousness Project: This international collaboration maintains a network of random number generators around the world and analyzes whether their outputs show non-random patterns during major world events that capture widespread human attention. Analysis of data from over 500 events has shown small but statistically significant deviations from randomness, suggesting possible field effects of collective consciousness. These findings support the Poia concept of consciousness as a field phenomenon that can interact with quantum systems.
Presentiment Studies: Research on presentiment—physiological responses that precede unpredictable stimuli—suggests that consciousness may interact with quantum systems across conventional temporal boundaries. Meta-analyses by researchers such as Dean Radin and Julia Mossbridge have found small but consistent effects across multiple laboratories and protocols. These findings align with the Poia proposal that consciousness operates non-linearly with respect to time, potentially influencing quantum systems through retrocausal effects.
Double-Slit Consciousness Experiments: Studies by Dean Radin and colleagues have tested whether conscious observation affects interference patterns in double-slit experiments without physical interaction. Their results suggest small but measurable effects of conscious attention on quantum wave function collapse. While these findings require further replication and validation, they directly test the consciousness-collapse mechanism proposed by the Poia Theory.
While these consciousness-quantum interaction studies remain controversial within mainstream science, their consistent results across different laboratories, experimenters, and protocols provide suggestive evidence that consciousness may indeed influence quantum processes as proposed by the Poia Theory.
4. Brain-Consciousness Correlation Studies
Research examining correlations between brain states and consciousness provides indirect support for quantum models:
Gamma Synchrony Research: Studies have consistently found correlations between consciousness and gamma-band (30-90 Hz) synchronization across the brain. This synchronization occurs at frequencies that match predictions from quantum models of consciousness, including the Orch-OR theory that the Poia Theory builds upon. The specific patterns of this synchronization suggest quantum-like integration of information across the brain.
Meditation Neuroimaging: Research on advanced meditation practitioners has identified unique brain states associated with expanded consciousness experiences. Studies using EEG, fMRI, and other techniques have found patterns of neural coherence and integration that align with predictions from quantum models of consciousness. These findings support the Poia proposal that certain brain states facilitate stronger coupling with the consciousness field through enhanced quantum coherence.
Psychedelic Neuroimaging: Studies of psychedelic substances that alter consciousness have found increased neural entropy and connectivity patterns consistent with expanded quantum coherence. Research using various neuroimaging techniques has shown that psychedelics create brain states characterized by increased information integration and reduced constraint from predictive models. These findings align with the Poia understanding of how certain brain states may enhance quantum-consciousness interactions.
Near-Death Experience Research: Studies of near-death experiences (NDEs) have documented cases where individuals report veridical perceptions during periods of minimal brain activity. While methodologically challenging to study, these cases suggest that consciousness may not be entirely dependent on classical brain activity, potentially supporting field models of consciousness that interact with the brain through quantum processes.
These brain-consciousness correlation studies provide indirect support for quantum models by identifying neural patterns consistent with quantum-consciousness interactions and cases where consciousness appears to transcend classical neural constraints.
Methodological Challenges and Responses
Research on quantum aspects of consciousness faces several significant methodological challenges:
1. Measurement Challenges
Directly measuring quantum effects in living neural tissue presents enormous technical difficulties:
Quantum effects typically require extremely sensitive measurement techniques that may damage living tissue
The warm, wet environment of the brain creates noise that can mask quantum signals
Quantum effects may be distributed across scales and structures, making them difficult to isolate
Methodological Responses:
Development of non-invasive quantum sensing technologies such as nitrogen-vacancy diamond sensors
Creation of experimental models that bridge between isolated quantum systems and living neural tissue
Application of advanced signal processing techniques to extract quantum signals from biological noise
Design of experiments that test for quantum effects through their macroscopic consequences rather than direct measurement
2. Replication Challenges
Consciousness-related effects may be sensitive to factors not typically controlled in physical experiments:
Experimenter consciousness may influence results in ways difficult to control
Participant psychological factors may affect quantum interactions
Environmental and contextual variables may influence consciousness-quantum interactions
Statistical power issues may arise from small effect sizes
Methodological Responses:
Development of standardized protocols that specify both physical and consciousness-related variables
Implementation of automated systems that minimize direct experimenter involvement
Pre-registration of experimental designs and analysis plans to prevent post-hoc adjustments
Conduct of large-scale, multi-site replications with sufficient statistical power
Exploration of factors that may enhance effect sizes to improve replicability
3. Theoretical Integration Challenges
Interpreting experimental results requires integrating findings across disciplines that often use different terminology and frameworks:
Physics, neuroscience, and consciousness studies use different languages and concepts
Theoretical frameworks for quantum-consciousness interactions remain incompletely formalized
Conventional statistical approaches may not adequately capture quantum probability effects
Methodological Responses:
Development of interdisciplinary research teams with expertise across relevant fields
Creation of formal mathematical models that bridge quantum physics and consciousness studies
Application of quantum probability theory rather than classical statistics when appropriate
Establishment of common terminology and conceptual frameworks across disciplines
Future Research Directions
Based on current evidence and the predictions of the Poia Theory, several promising research directions emerge:
1. Advanced Quantum Measurements in Neural Systems
Developing more sophisticated technologies and methodologies for detecting quantum effects in neural systems:
Specific Research Approaches:
Nitrogen-Vacancy Diamond Sensors: These quantum sensors can detect magnetic fields with extraordinary sensitivity and spatial resolution. They could potentially measure quantum coherence in neural structures non-invasively by detecting the magnetic signatures of quantum processes.
Quantum-Enhanced Microscopy: Techniques such as quantum optical coherence tomography could potentially visualize quantum coherence in living neural tissue with unprecedented resolution, potentially revealing quantum processes in structures like microtubules.
Biophoton Detection Arrays: Advanced systems for detecting and characterizing the quantum optical properties of biophotons emitted by neural tissue could map quantum optical networks in the brain and their correlation with neural activity and consciousness states.
Quantum Resonance Spectroscopy: Techniques for measuring quantum resonance patterns across multiple frequency bands could characterize the quantum properties of neural structures and their changes during different consciousness states.
Expected Outcomes: These approaches could provide direct evidence for quantum processes in neural systems, characterize their properties and distribution, and establish correlations between neural quantum states and consciousness states.
2. Consciousness-Quantum Interaction Studies
Designing rigorous experiments to test whether and how consciousness influences quantum systems:
Specific Research Approaches:
Advanced Double-Slit Experiments: Refined versions of consciousness-influenced double-slit experiments with stronger controls, larger sample sizes, and variations in consciousness conditions could provide more definitive tests of consciousness-collapse interactions.
Quantum Random Number Generator (QRNG) Influence: Experiments testing whether specific consciousness states or intentions can influence the output of quantum random number generators could be conducted with pre-registered protocols, automated systems, and large sample sizes to maximize evidential value.
Resonance-Based Quantum Influence: Studies testing whether intention effects on quantum systems are stronger when the intention is "tuned" to resonate with the system's characteristics could test the resonance mechanism proposed by the Poia Theory.
Collective Consciousness Quantum Effects: Experiments comparing the effects of individual versus group consciousness on quantum systems could test the Poia prediction that collective consciousness creates stronger field effects through constructive interference.
Expected Outcomes: These studies could establish whether consciousness genuinely influences quantum systems, characterize the conditions that enhance or inhibit these effects, and test specific mechanisms proposed by the Poia Theory such as resonance and collective field effects.
3. Neural-Quantum Correlation Studies
Investigating correlations between quantum processes in the brain and consciousness states:
Specific Research Approaches:
Anesthetic Quantum Effects: Studies examining how different anesthetics affect quantum coherence in neural structures could establish more direct links between quantum brain processes and consciousness, potentially identifying which quantum properties are most relevant to consciousness.
Meditation Quantum Effects: Research on how meditation practices affect quantum coherence in the brain could test whether consciousness practices enhance quantum-consciousness coupling as predicted by the Poia Theory.
Psychedelic Quantum Effects: Studies of how psychedelic substances affect neural quantum coherence could provide insights into how these consciousness-altering compounds might work through quantum mechanisms.
Sleep and Dream Quantum Correlates: Research examining quantum processes during different sleep stages and dream states could reveal how consciousness states correlate with quantum brain dynamics across the sleep-wake cycle.
Expected Outcomes: These studies could establish specific correlations between consciousness states and neural quantum processes, identify which quantum properties are most relevant to consciousness, and test whether practices that alter consciousness do so through quantum mechanisms.
4. Quantum Biology of Microtubules
Deeper investigation of the quantum properties of microtubules and their relationship to consciousness:
Specific Research Approaches:
Microtubule Quantum Coherence Mapping: Studies using advanced measurement techniques to map quantum coherence in microtubules under various conditions could characterize their quantum properties and how they are affected by factors relevant to consciousness.
Tubulin Quantum Simulations: Computational models simulating the quantum properties of tubulin proteins and microtubule assemblies could predict their quantum behavior and guide experimental investigations.
Microtubule-Biophoton Interactions: Research examining interactions between microtubules and biophotons could test whether microtubules function as quantum optical devices that process information through light.
Pharmacological Quantum Modulation: Studies of how various compounds affect microtubule quantum properties could identify substances that enhance or inhibit quantum coherence, potentially leading to new tools for consciousness research and applications.
Expected Outcomes: These studies could establish the specific quantum properties of microtubules, how they are maintained in living neurons, and how they correlate with consciousness, potentially confirming their proposed role as quantum processors relevant to consciousness.
5. Quantum-Classical Transition in Neural Systems
Investigating how quantum effects in the brain influence classical neural activity:
Specific Research Approaches:
Scale-Bridging Studies: Research examining how quantum effects at smaller scales influence classical processes at larger scales could identify the specific mechanisms through which quantum processes affect neural activity.
Criticality and Quantum Effects: Studies of how neural criticality—the state between order and chaos where the brain appears to operate—relates to quantum processes could reveal how quantum effects might be amplified through critical dynamics.
Quantum Noise Influence: Research on how quantum fluctuations influence the noise characteristics of neural systems could establish whether quantum indeterminacy plays functional roles in neural processing.
Temporal Bridging Mechanisms: Studies examining how processes at quantum timescales influence events at classical neural timescales could identify the specific temporal bridging mechanisms that connect quantum and classical domains.
Expected Outcomes: These studies could establish the specific pathways through which quantum effects influence classical neural activity, the conditions that enhance or inhibit these influences, and the functional significance of quantum-classical interactions in neural processing.
6. Collective Consciousness Field Studies
Investigating potential field effects of collective consciousness:
Specific Research Approaches:
Global Field Consciousness Networks: Expanded versions of the Global Consciousness Project with more sophisticated quantum sensors and analysis methods could better characterize potential field effects of collective consciousness during major events.
Synchronized Meditation Studies: Research on quantum coherence effects during synchronized meditation by multiple individuals could test whether collective consciousness creates stronger field effects than individual consciousness.
Field Consciousness Propagation: Studies examining how consciousness field effects might propagate through space and across individuals could characterize the field properties of consciousness proposed by the Poia Theory.
Environmental Quantum Correlations: Research on correlations between human consciousness and quantum processes in the environment could test whether consciousness fields interact with environmental quantum systems as predicted by the Poia Theory.
Expected Outcomes: These studies could establish whether collective consciousness creates field effects that influence quantum systems, characterize the properties and extent of these fields, and test specific predictions of the Poia Theory regarding collective consciousness phenomena.
7. Practical Applications Development
Developing technologies and methodologies based on quantum-consciousness interactions:
Specific Research Approaches:
Consciousness-Enhanced Quantum Technologies: Research on whether specific consciousness states or intentions can enhance the performance of quantum computing, quantum communication, or quantum sensing technologies could lead to hybrid systems that leverage consciousness-quantum interactions.
Quantum-Based Consciousness Assessment: Development of technologies that measure neural quantum coherence as indicators of consciousness states could provide new tools for consciousness research, medical applications, and personal development.
Quantum Resonance Healing: Research on whether intention directed through quantum resonance principles can influence biological systems could develop more effective approaches to consciousness-based healing.
Quantum-Consciousness Interface Devices: Development of technologies that facilitate more direct interaction between consciousness and quantum systems could create new forms of human-machine interface based on quantum principles.
Expected Outcomes: These applied research directions could develop practical technologies and methodologies that both utilize and provide evidence for quantum-consciousness interactions, potentially creating new fields at the intersection of quantum technology and consciousness studies.
Integration with Broader Scientific Frameworks
For the Poia Theory to gain broader scientific acceptance, research must demonstrate how its proposals integrate with established scientific frameworks:
1. Integration with Neuroscience
Research showing how quantum brain processes complement rather than contradict established neuroscience:
Studies demonstrating how quantum effects in neural structures influence conventional neural signaling
Research showing how quantum and classical information processing might work together in the brain
Investigations of how quantum proposals can address explanatory gaps in classical neuroscience
2. Integration with Physics
Research establishing connections between consciousness studies and fundamental physics:
Theoretical work formalizing how consciousness might interact with quantum fields without violating physical laws
Experimental tests of specific physical mechanisms for consciousness-matter interactions
Studies exploring potential connections between dark matter and consciousness fields
3. Integration with Evolutionary Biology
Research examining the evolutionary aspects of quantum-consciousness interactions:
Studies of quantum effects across species with different neural complexity
Investigations of how quantum-consciousness interactions might provide evolutionary advantages
Research on the co-evolution of quantum biological mechanisms and consciousness
Conclusion: An Evidence-Based Path Forward
The experimental evidence supporting quantum aspects of consciousness, while not yet conclusive, provides sufficient foundation to justify serious scientific investigation of the proposals in the Poia Theory of Everything. The convergence of findings from quantum biology, neuroscience, physics, and consciousness studies suggests that quantum processes may indeed play important roles in consciousness, potentially serving as the interface through which consciousness interacts with physical reality.
The future research directions outlined here offer concrete paths for testing and developing the Poia Theory through rigorous scientific methodology. By generating specific, testable predictions and engaging with existing scientific frameworks, the theory demonstrates that even revolutionary concepts about consciousness and reality can be approached through evidence-based scientific inquiry.
As these research programs advance, they may not only test the specific proposals of the Poia Theory but contribute to a broader scientific understanding of consciousness and its relationship to physical reality. Whether the theory ultimately proves correct in all its aspects or requires significant revision, the pursuit of these research directions promises to deepen our understanding of one of the most profound questions in science: how consciousness relates to the physical world.
This evidence-based approach honors both the revolutionary potential of the Poia Theory and the empirical foundation of scientific inquiry, creating a path forward that neither dismisses unconventional ideas nor accepts them without rigorous testing. It is through this balanced approach that genuine scientific progress occurs, potentially leading to paradigm shifts that transform our understanding of consciousness, quantum physics, and the fundamental nature of reality itself.
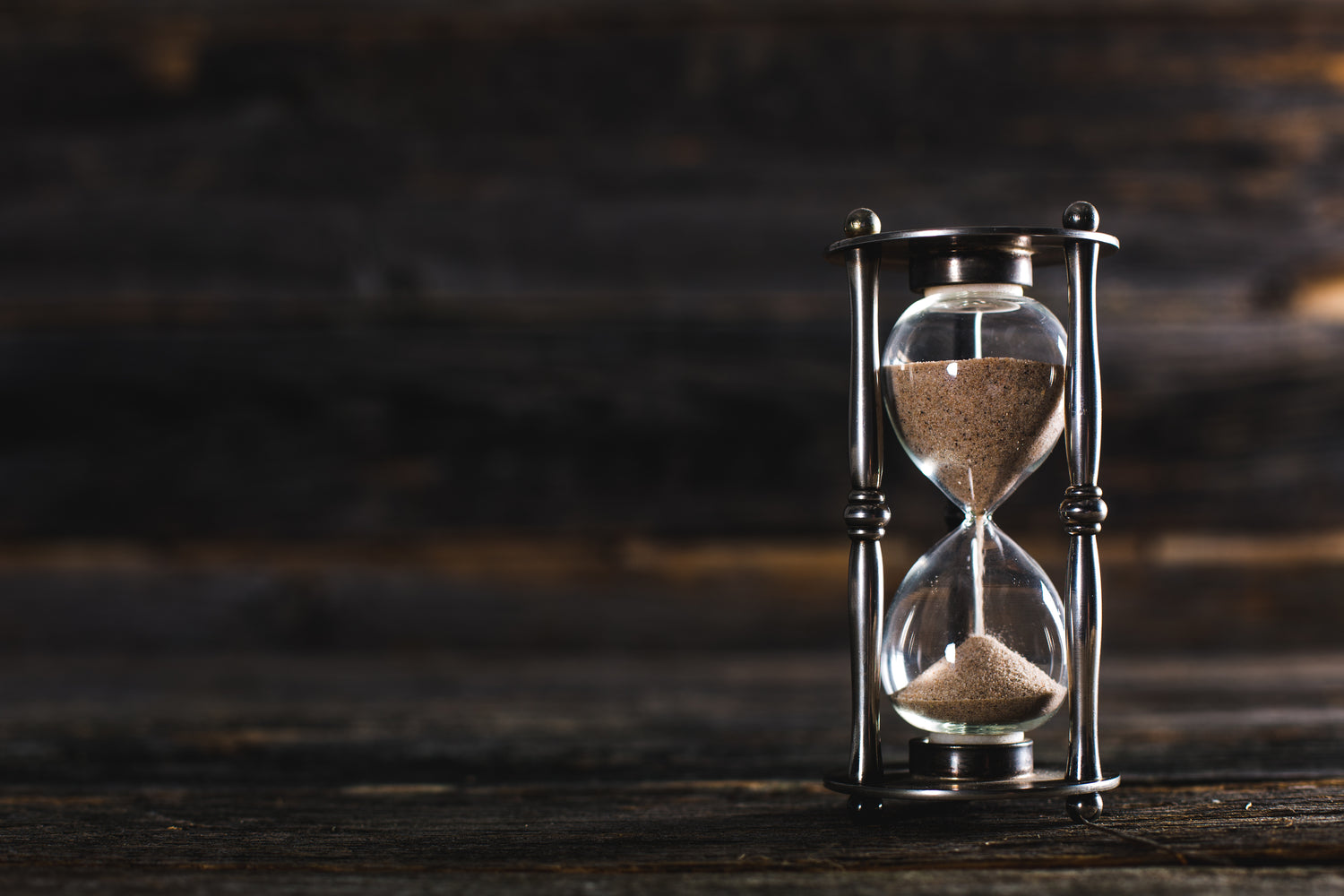
Time
Pair text with an image to focus on your chosen product, collection, or blog post. Add details on availability, style, or even provide a review.