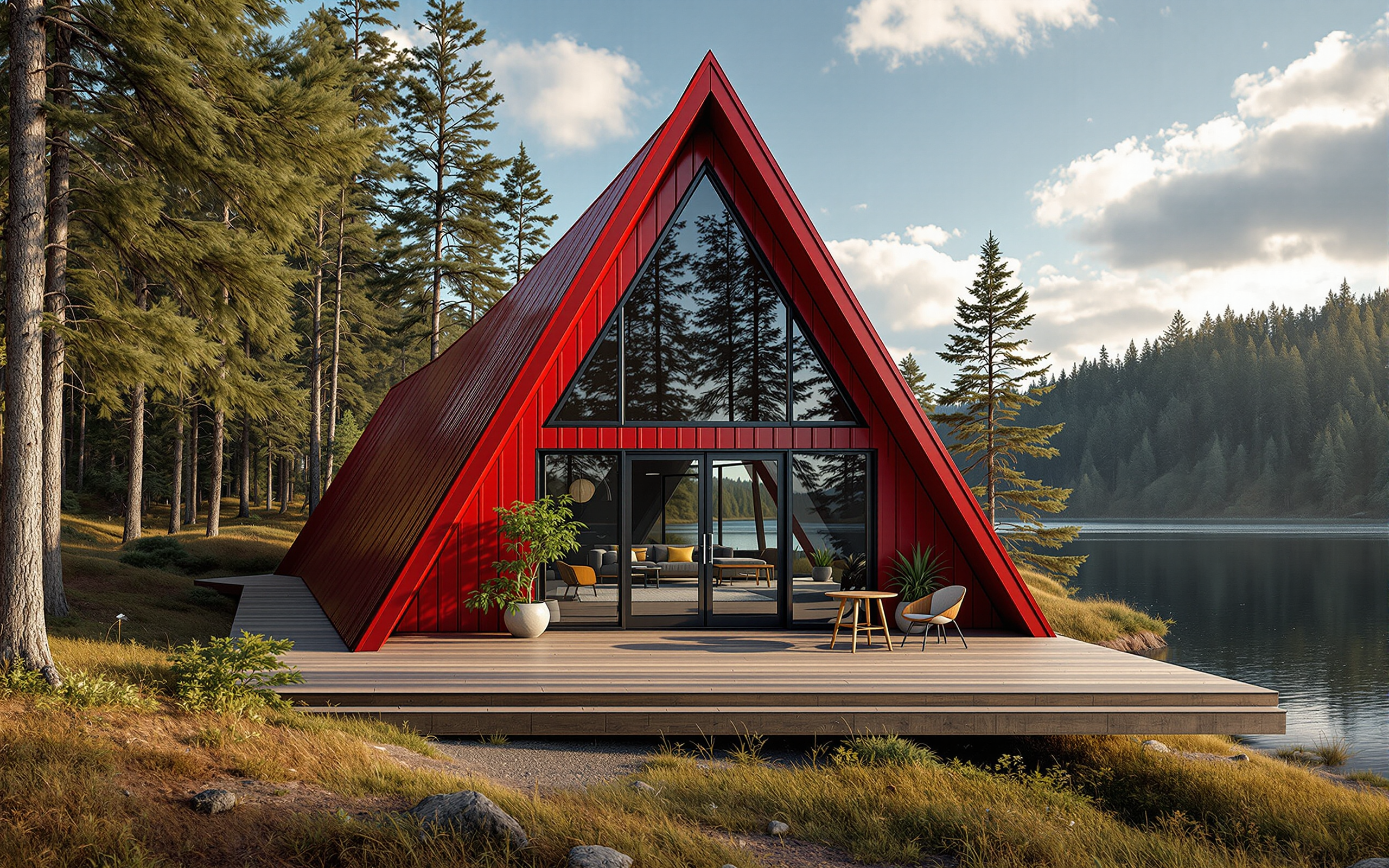
The Self Lens - Chapter 10
Consciousness in a Hilbert Space Framework
In the Self Lens model, a conscious mind is envisioned as a quantum system, meaning its states can be represented as vectors in an abstract Hilbert space. A Hilbert space is a mathematical space of (possibly infinitely many) dimensions with an inner product, providing the stage on which quantum states “live.” In this framework, each possible conscious state – encompassing all aspects of one’s subjective experience at an instant – corresponds to a vector (often denoted |Ψ⟩) in a very high-dimensional Hilbert space. The sheer dimensionality reflects the enormous complexity of consciousness: countless degrees of freedom (from sensory inputs to memories and thoughts) contribute to the full state. Formally, we might say an individual’s state at time t is |Ψ(t)⟩ ∈ H, where H is the Hilbert space of all their possible experiences. This state can be thought of as a superposition (a weighted sum) of basis vectors, each basis vector representing a particular definite conscious configuration (for example, a specific perception or thought pattern). Mathematically, one could write:
∣Ψ(t)⟩=∑_nc_n(t) ∣n⟩,|Ψ(t)\rangle = \sum\_n c\_n(t)\, |n\rangle,
where {|n⟩} is some basis spanning the conscious-state space (each |n⟩ might correspond to an elementary experiential state), and the complex coefficient ()c_n(t)() tells us the amplitude (and phase) of that component in the mind’s state at time t. Because the coefficients are generally complex numbers, the state |Ψ⟩ carries phase information as well as intensity for each configuration. The inner product structure of Hilbert space allows us to define overlaps or “similarities” between states – e.g. ⟨Φ|Ψ⟩ – which in this context could measure how similar two mind-states are (though defining a concrete basis for consciousness is a deep challenge, conceptually).
Adopting the Hilbert space formalism grants us a powerful language: we can apply the well-developed machinery of quantum mechanics to consciousness. For example, we can consider operators on this space that correspond to observable aspects of consciousness. In physics, an operator (like a Hamiltonian or a spin operator) acts on state vectors and has eigenvalues corresponding to measurement outcomes. By analogy, we could introduce operators for various cognitive observables. The Self Lens model in its formal development does exactly this: it defines, for instance, an “identity operator” ()\hat{I}() whose eigenstates represent well-defined senses of self, or an “intention operator” ()\hat{Int}() capturing directed goals. Observables like these are speculative – real neuroscience hasn’t identified such precise operators – but they serve as useful placeholders to discuss what measuring a certain aspect of consciousness would entail in the quantum view. If one were to measure the “awareness operator” ()\hat{A}() on the state |Ψ⟩, the outcome might correspond to a reported level of mindfulness or clarity at that moment, with the state collapsing into an eigenstate of ()\hat{A}() (much as measuring a spin-()\frac{1}{2}() particle’s spin-()z() yields an eigenstate either “up” or “down”).
Crucially, the quantum formalism treats the state |Ψ⟩ as fundamentally uncertain or distributed over possibilities until a measurement occurs. In a consciousness context, this suggests that prior to “deciding” or “observing” a particular experience, the mind could exist in a blend of potential experiences. This is not the everyday way we think of our mind – we usually feel we have a single definite experience at a time – but the Hilbert space perspective encourages us to consider the underlying potential states that vie to become the moment-to-moment conscious reality. The superposition principle implies that the mind can, in principle, hold incompatible or contrasting perceptions simultaneously in a quantum sense, though only one will be realized when observed (analogous to a quantum particle being in a superposition of locations until one position is measured). In the Self Lens model’s terms, consciousness might explore a space of possibilities (superposed mind states) before “collapsing” to a specific experience via attention or observation.
Because the Hilbert space of consciousness is likely vast, it may be useful to consider simplified subspaces or effective qubits for illustration. For instance, one might model a binary cognitive variable (like a simple decision “Yes” or “No”) as a two-dimensional Hilbert subspace spanned by |Yes⟩ and |No⟩. Any undecided or ambivalent state could be a superposition ()a|Yes\rangle + b|No\rangle(), which parallels the use of qubits in quantum computing. Indeed, researchers in the field of quantum cognition have successfully used low-dimensional Hilbert spaces to model human decision-making and concept association, not implying the brain is literally a quantum computer, but that the probability mathematics of quantum theory can describe how humans violate classical logic expectations. In those models, the complex phase and interference of cognitive “amplitudes” can explain phenomena like changes of preference based on question order, or uncertainty in decision, which classical probability struggles with. The Self Lens model’s ambitions go further – suggesting that consciousness is not just modeled by quantum mathematics, but may physically instantiate a quantum process. Under that assumption, the Hilbert space representation becomes more than analogy: it would be a state-space for an actual quantum system (the brain or the mind) with an extremely rich structure.
Phase Coherence and Superposed Mind States
Within the Hilbert space formulation, the notion of phase coherence becomes central to understanding how different components of a conscious state relate. Coherence in quantum mechanics refers to the maintenance of well-defined phase relationships between parts of a superposed state. If two components of a wavefunction have a fixed phase relationship, they can interfere – leading to phenomena like the interference pattern in the double-slit experiment. By analogy, if different aspects of a person’s mind (different neural or cognitive processes) remain phase-coherent with each other, the system can exhibit interference-like effects that would have no classical counterpart. In practical terms, quantum phase coherence would allow disparate mental subprocesses to combine constructively or destructively. Constructive interference between components of |Ψ⟩ might amplify certain mental contents (making a perception or idea dominant), whereas destructive interference might cancel others out, potentially corresponding to suppression or resolution of ambiguity.
For example, consider a simplified superposition |Ψ⟩ = ()c_1|α\rangle + c_2|β\rangle() of two brain states representing two interpretations of an ambiguous image (like the famous face/vase optical illusion). If the phases of ()c_1() and ()c_2() are coherent, the interference between the “face” perception and the “vase” perception could lead to rapid switching or a mixed percept, depending on how the observation is made. If something disrupts the phase coherence (more on decoherence later), the state may effectively randomize into a classical mixture (with some probability the brain is in the face state, some in the vase state, but not a coherent superposition). Maintaining coherence across neural assemblies might then be key to any quantum advantage in cognition, such as the ability to consider multiple mutually exclusive hypotheses or perceptions simultaneously in a “superposed” way. If the brain can hold such superpositions, it could in principle perform a kind of parallel evaluation of possibilities akin to quantum parallelism in computation. Only when a choice or definite perception is needed would the superposition collapse, yielding a single conscious outcome (the face or the vase). This line of thought resonates with the Self Lens model’s idea that consciousness spans a field of possibilities and only concretizes reality upon “looking” (i.e., upon a reflective act of awareness).
Phase coherence might also be related to the unity of consciousness. One longstanding puzzle is how diverse sensory inputs and processes unify into a single coherent subjective experience (the “binding problem”). In classical neuroscience, this is sometimes attributed to synchronized neuronal firing or oscillations (e.g., gamma oscillations linking different brain regions). In the quantum view, one could speculate that the brain maintains a globally entangled state – a coherent superposition that encompasses numerous subsystems (visual cortex, auditory cortex, etc.). The phase alignment of the wavefunction parts ensures these subsystems are not independent but parts of one holistic state. As long as coherence is preserved, the different modalities can exchange information in non-trivial ways (not necessarily signaling in the classical sense, but by virtue of being components of the same quantum state). For instance, the phase of neural oscillations might even modulate or be modulated by underlying quantum-coherent processes; if neurons or micro-structures (like dendritic protein states or microtubules) support coherent superpositions, their collective phase could sync up, enabling cross-modal interference.
It’s important to stress that the existence of such large-scale quantum coherence in the brain is highly speculative. The warm, wet environment of neural tissue is usually considered hostile to quantum coherence, as random interactions (with water molecules, ions, etc.) tend to disturb phase relationships extremely quickly. In quantum physics terms, the brain is an open system constantly interacting with its environment (including internal thermal vibrations), which ordinarily causes rapid decoherence. Nevertheless, some theorists (notably in quantum brain research) have proposed mechanisms to sustain micro-scale coherence. For example, photosynthetic complexes in biology manage to preserve quantum coherence for surprisingly long times at ambient temperatures to efficiently transfer energy. Analogously, it has been hypothesized that certain structures in neurons (like microtubules or ion channels) might have special properties (quantum error-correction, shielding by ordered water, etc.) that let them retain coherence longer than naive estimates suggest. If such coherence can last even on the order of microseconds (as some optimistic analyses claim, in contrast to far shorter femtosecond scales from pessimistic calculations, it could conceivably be relevant for neural processes which operate on the millisecond timescale. While the jury is still out, the Self Lens model assumes for the sake of exploration that at least some coherent quantum processes contribute to conscious dynamics, thereby making phase coherence a meaningful concept for consciousness. Under that assumption, consciousness might be viewed as a self-coherent field, where the self (the “observer” in the model’s parlance) effectively phase-locks certain aspects of the brain’s quantum state into alignment, ensuring a stable experience arises from the underlying superposition.
Mathematically, coherence is described by the off-diagonal elements of a density matrix ρ. If ρ = |Ψ⟩⟨Ψ| is a pure state, it has maximal coherence relative to itself. But if the state becomes a mixture ρ = ∑_i p_i |Ψ_i⟩⟨Ψ_i| (with probabilities p_i for different basis states, as would happen after decoherence), the off-diagonal terms (⟨Ψ_i|ρ|Ψ_j⟩ for i≠j) drop toward zero. Those off-diagonals carry the phase relationships. In a brain context, losing those terms means the different possible mind-states in the mixture no longer interfere or superpose; the system behaves as if it is in one definite state at a time (just not a single predictable one – it’s randomly one of the Ψ_i with probabilities p_i). Thus, phase coherence is the mathematical key to a genuine quantum-conscious state that isn’t just a classical lottery of states but a live superposition of them. The Self Lens model’s quantum stance implies that consciousness crucially hinges on maintaining such off-diagonal coherence at least within some core parts of the system, so that the self can “view” multiple potentials simultaneously before collapsing one into reality.
Quantum Measurement and Conscious Observation
The act of measurement plays a dual role in a quantum model of consciousness. First, there is the idea (dating back to von Neumann and Wigner) that consciousness itself might be the ultimate measurement apparatus – that a conscious observation causes the collapse of the quantum wavefunction into a definite state. Second, within the Self Lens model, we can also consider consciousness as a system that performs measurements on itself and on its environment. Both perspectives involve the formalism of quantum measurement theory: operators with eigenstates, projection postulates, probabilities given by Born’s rule, and so on.
In standard quantum mechanics, a measurement is an interaction that entangles a quantum system with a measuring device, and ultimately yields a single outcome, seemingly at random (with probability given by the wavefunction’s amplitudes squared). If we treat the mind-brain as the quantum system of interest, what is the measuring device and what constitutes an outcome? One view (aligned with some interpretations of quantum mechanics) is that conscious awareness itself is not just a passive observer but actively reduces the state – that when you “become aware” of a thought or a perception, that very act is analogous to a quantum measurement collapsing a superposition of possible thoughts into one actual thought. In this interpretation, prior to conscious awareness, the brain might carry multiple latent possibilities (e.g. competing interpretations, multiple possible action plans) in a quantum superposition or indefinite state. The moment of conscious recognition or decision is the measurement event that crystallizes one possibility into experience. This has a philosophical appeal of potentially addressing the “observer” problem in quantum physics – rather than requiring an external classical device, the chain of measurements (Wigner’s friend style) ends at consciousness which itself is fundamental. However, it’s also controversial, because it ascribes a special role to consciousness that is outside the unitary Schrödinger evolution, effectively saying that the mind causes collapse (a stance associated historically with physicist John von Neumann and later Eugene Wigner’s speculations).
The Self Lens model tends toward a monist view (consciousness as fundamental), so it entertains the notion that conscious observation is indeed a special kind of measurement that selects real outcomes from quantum possibilities. For example, if the state |Ψ⟩ includes a superposition of intention outcomes (say one part of the state intends to raise the left hand, another intends to raise the right hand), the action of actually doing one or the other corresponds to the conscious will “measuring” and collapsing the intention operator ()\hat{Int}(), yielding a definite intention that then translates to motor output. In formal terms, if ()\hat{Int}() has intention eigenstates |Left⟩ and |Right⟩, an initial state ()a|Left⟩ + b|Right⟩() will, upon measurement, collapse to |Left⟩ with probability |a|² or to |Right⟩ with probability |b|², matching the Born rule. The outcome is now a classical decision – the superposition is gone post-measurement. This random element might sound at odds with our sense of deliberate choice, but in a quantum model some of what we consider free will could involve stochastic processes or biases at the quantum level (some proponents of quantum mind have even suggested true randomness from quantum events could inject indeterminism into our choices, aligning with a sort of libertarian free will – though this is a philosophical can of worms).
Apart from consciousness causing measurement, we can also flip the perspective to consciousness as the system measured by external devices. For instance, when a neuroscientist uses an EEG or fMRI, they are effectively measuring some observables of the brain (like electromagnetic fields, or metabolic activity). Those measurements collapse certain aspects of the brain’s state (though these macroscopic measurements are well in the classical regime due to decoherence, so this is not exotic collapse, just classical observation of a classicalized quantity). But even internally, different parts of the brain could be “measuring” each other. Neurons connecting to other neurons perform a kind of measurement – they sense signals (neurotransmitter release either happened or not) and respond. At the quantum level, if microstates of one part of a neuron are entangled with another, an interaction could entangle and effectively measure a particular basis. Thus, we can imagine a hierarchy of measurements cascading in the brain: small-scale events (like a protein changing conformation) might collapse some micro-superposition; larger-scale events (like a neuron firing) might be seen as measuring an aggregate state of many proteins or ions; and so on, up to the level of whole brain assemblies. Ultimately, global brain measurements could correspond to moments of unified conscious experience. In other words, the brain might constantly measure itself, but only certain large-scale, system-wide measurement events register as conscious awareness in the Self Lens view.
This view ties into the concept of the von Neumann chain in quantum measurement theory – typically, when a quantum system is measured by a device, that device becomes entangled and, if not observed, you have a bigger entangled state (system+device). You need another observer to measure the device, and so on. If one posits consciousness as the terminus of this chain, it suggests consciousness doesn’t need an external observer; it is the observer that collapses the chain. In the brain, one could imagine nested interactions from the molecular scale up to the neural network scale; if these interactions ultimately culminate in a state that interfaces with consciousness (the Self), that final interaction could be where the collapse effectively happens, yielding a reportable experience. The challenge is describing where and how this occurs in physical terms, which remains an open question.
Mathematically, modeling measurement within the system might involve certain operators (like those ()\hat{I}, \hat{A}, \hat{Int}() mentioned) and treating the self as a special subsystem that interacts with others. One could conceive of the “self” as an internal observer – perhaps a mode of the brain’s quantum state that has a persistent existence and entangles with various content. When this self mode entangles strongly with a particular content component (say an experience of red color), that entanglement could effectively collapse the rest of the brain’s state relative to that content (decohering alternatives). Some interpretations of quantum mechanics, like the relative state (Many-Worlds) interpretation, would frame this as the self becoming correlated with that content in one branch of the wavefunction. The Self Lens model, however, seems to favor a single-chain collapse (since it speaks of consciousness creating reality), so it would assert that alternative branches do not continue to exist once the self has observed a particular outcome – there is one experienced reality stream.
In summary, quantum measurement theory provides a language to discuss how conscious experiences become definite. It requires clearly defined observables and a collapse mechanism. The Self Lens model posits consciousness both as a process that collapses quantum states and as a state that can be analyzed in quantum terms – a somewhat paradoxical but intriguing dual role. Future theoretical development of this model could borrow from the frameworks of quantum measurement in continuous systems (since brain dynamics are ongoing, not single isolated measurements) and decoherence theory to see how classical experience emerges from underlying quantum possibilities.
Weak Measurements and Partial Awareness
Not all measurements need to be all-or-nothing affairs. In quantum physics, there is a concept of weak measurement where one gently probes a system, acquiring a small amount of information without forcing it into an eigenstate. After a weak measurement, the system is disturbed only slightly and retains much of its prior coherence. If consciousness operates in a quantum regime, one might wonder if the brain sometimes performs partial observations of its internal state – akin to weak measurements – and whether this could correspond to states of partial awareness or preconscious processing.
In a weak measurement, the outcome is not a definite eigenvalue but rather an averaged shift in the measuring device, from which an inferred quantity called a weak value can be obtained. Interestingly, weak values can sometimes lie outside the range of eigenvalues of the measured observable, and they reflect the peculiar interference of pre- and post-selected quantum states. Translating this to a cognitive scenario: suppose the mind has not fully decided on a perception or a concept (no strong measurement yet), but some internal processes “peek” at the evolving superposition state. This could be analogous to having a hunch or intuition about something without full clarity. The brain might register an ambiguous, exaggerated, or context-dependent signal – reminiscent of a weak value – that guides further processing. For example, when solving a problem, you might have a vague intuition of the direction to go (a sense “the answer is going to be a large number” or “I recall something like X might be relevant”) even before the solution is fully formed. One could speculate that this intuition is the result of a weak measurement on the superposed thoughts or memories in the brain, giving a “weak value” that hints at the eventual outcome without collapsing all possibilities outright.
Another area where the idea of weak measurement could apply is subliminal perception. Experiments show that stimuli presented very briefly or masked can influence us (priming effects) even if we aren’t consciously aware of them. In a quantum mind picture, perhaps the stimulus is registered in the brain’s state (becoming a component of |Ψ⟩) but isn’t strongly measured by the “self” system. It’s as if the conscious self only weakly coupled to that stimulus – enough to tilt some biases (like faster reaction to related words), but not enough to produce a clear conscious perception. The result is an unconscious or barely conscious influence. This is analogous to a weak measurement where the system (brain) picks up some information about the stimulus without full collapse (no distinct conscious perception). The weak value here might manifest as an unconscious tendency or a feeling without a known source.
Formally, one can model a weak measurement by coupling the system to a meter with a very small interaction strength. The system’s state |Ψ⟩ then only slightly “collapses” and mostly continues in a smeared superposition. If the brain employs such gentle probing internally – perhaps via neural feedback loops that are sensitive but not overwhelming – it could preserve coherence longer while still extracting useful information gradually. This could be advantageous: the brain might iteratively refine the state by successive weak measurements, each time narrowing down the possibilities without a full collapse until sufficient evidence accumulates for a strong, conscious measurement (decision or perception). Such a process would be a quantum sequential sampling of information. It aligns in spirit with how some cognitive models describe decision-making as gradually accumulating evidence until a threshold – here the twist is that evidence accumulation might occur through quantum interference and weak observations of latent states.
Though highly theoretical, if any of this is true, one might find traces by looking for effects predicted by quantum measurement theory in psychological experiments. One possible avenue is checking Leggett–Garg inequalities in cognitive dynamics, which are inequalities that classical systems (with defined states at all times) obey, but quantum systems can violate. A violation of a Leggett–Garg inequality in, say, human decision dynamics (as some researchers in quantum cognition have considered) would indicate that our choices cannot be modeled as having definite predetermined states at all times – consistent with the idea of superposition and perhaps weakly probing them over time. While no conclusive violation has been detected in humans yet, the framework to test such things is being explored in the quantum cognition community.
In essence, weak measurements extend the Self Lens model by suggesting consciousness need not be an all-or-nothing observer. The self can “peek” at itself in flight, so to speak. This enriches the picture of conscious processing: alongside moments of clarity (strong measurement, collapse to a definite experience), there may be periods of hazy, tentative awareness (weak observations) where multiple possibilities coexist and subtly influence our leanings. It’s a nuanced quantum nuance to the model, highlighting that even observation can be graded and gentle, not just a binary collapse.
Decoherence: From Quantum Potential to Classical Perception
No discussion of quantum consciousness is complete without addressing decoherence, the process by which quantum systems lose their coherence and begin to behave classically due to interaction with their environment. Decoherence is often seen as the principal enemy of any quantum effects in the brain, as the brain at body temperature is swarming with particles, photons, and fluctuating fields that can carry away the crucial phase information. When a quantum system decoheres, it for practical purposes undergoes an apparent wavefunction collapse (even though in some interpretations, the wavefunction of system+environment is just becoming entangled). The result is that the system can be described as a statistical mixture of states rather than a pure superposition. In the context of consciousness, decoherence is what would make the brain’s state effectively classical long before any putative quantum advantages could be realized, unless the model has a strategy to mitigate it.
The calculations by physicist Max Tegmark in 2000 famously estimated that neuron-level quantum states would decohere on the order of ()10^{-13}() to ()10^{-20}() seconds – trillionths of a second – astoundingly fast compared to neural firing times (~1-10 milliseconds). Even microtubule-level states were estimated to lose coherence in ()10^{-13}(). These estimates come from considering thermal collisions and electromagnetic interactions that would randomize phases. If these numbers are correct, any coherent superposition in the brain is snuffed out essentially instantaneously relative to cognition, making quantum effects negligible. In other words, by the time your brain could integrate quantum information, the delicate quantum state is already destroyed by the “hot, wet, and noisy” neural environment.
Proponents of quantum consciousness have responded to this challenge. Hameroff and colleagues, in the context of the Orch-OR theory, argued that Tegmark’s calculations were based on an overly pessimistic model of the microtubule quantum state separation. By considering smaller separations (femtometer-scale differences rather than tens of nanometers) and possible protective mechanisms (like shielding by ordered water or collective dipole oscillations), they obtained decoherence times maybe 10^6–10^7 times longer than Tegmark’s estimate. Even so, their revised coherence time was still far below the 25 milliseconds timescale of conscious events – perhaps in the microsecond range – but they introduce an additional twist: Orchestrated Objective Reduction (Orch-OR) posits that wavefunction collapse is driven by an objective quantum gravity mechanism at around 10–100 milliseconds for the orchestrated states in microtubules. In that model, rather than needing to maintain coherence that whole time, the system actively collapses (reduces) on a schedule governed by mass-energy differences (Penrose’s quantum gravity criterion). The collapse itself, orchestrated by cellular processes, would be the moment of conscious event. This is an ingenious way to circumvent pure environmental decoherence: essentially say that collapse (hence consciousness) happens before the environment fully decoheres the state, via a non-trivial quantum gravity effect. While Orch-OR remains speculative and controversial, it exemplifies the kind of solutions proposed to the decoherence problem – introducing new physics or special conditions to prolong or utilize quantum states.
Returning to a more general perspective, even if one doesn’t adopt Orch-OR specifically, the Self Lens model as a physical theory must account for how classical reality emerges from the underlying quantum substrate of consciousness. Decoherence theory provides that link. As the brain’s quantum state |Ψ⟩ interacts with the environment (which includes not just the external world but myriad internal degrees of freedom like surrounding cells, blood flow, etc.), the total state can be thought of as entangled:
∣Ψtotal(t)⟩=∑_ici∣ψi⟩brain⊗∣Ei⟩env,|\Psi_{\text{total}}(t)\rangle = \sum\_i c_i |ψ_i\rangle_{\text{brain}} \otimes |E_i\rangle_{\text{env}},
where {|ψ_i⟩} are brain states (e.g. different outcomes or thoughts) and |E_i⟩ are corresponding environment states that have “recorded” or become correlated with those brain states. If these environment states are nearly orthogonal (⟨E_i|E_j⟩ ≈ 0 for ()i \neq j()), the reduced state of the brain (obtained by tracing out the environment) becomes ()\rho_{\text{brain}} ≈ \sum_i |c_i|^2 |ψ_i\rangle\langle ψ_i|(), a classical mixture. At that point, for all practical purposes, the brain is in one of the ()|ψ_i⟩() with probability ()|c_i|^2(), and interference between the ()|ψ_i\rangle() terms is gone. This is essentially what happens when a conscious state becomes robustly classical – it can be viewed as having “chosen” an outcome. In daily life, most of our brain states are continually monitored by the environment (via sensory feedback, interactions, etc.), so they don’t remain in spooky superpositions.
Interestingly, decoherence can be a double-edged sword. On one hand, it fights against sustained quantum coherence; on the other, it allows a stable classical world to appear to us. If our brain states did not decohere, we might not experience a stable reality – we’d be in weird entangled limbo states with the environment. So even a quantum consciousness theory likely requires decoherence at some stage to have definite experiences. The key is to identify which interactions cause the collapse of which degrees of freedom and which degrees of freedom remain coherent (if any) to give quantum advantages. A quantum mind theory might say, for example, that micro-level variables (like certain electron spins or dipole orientations) remain coherent and entangled within parts of neurons to perform computations, but once the result is reached, it’s amplified to a classical neuron firing (decohering the result so it becomes definite and usable). This “amplification to classicality” is akin to how a quantum computer would ultimately need to produce a classical output that we read; the quantum bits must influence a measuring device that is classical. In the brain, neurons could act as such measuring devices for underlying quantum bits.
Another aspect to consider is decoherence and memory. If a conscious experience corresponds to a quantum state that later decoheres, how is it remembered? Likely the act of memory encoding itself requires decoherence – to store an event in synapses or neural circuits, those circuits must settle into a definite classical configuration representing the memory. Thus, each conscious moment (if quantum) must leave a classical trace to be recalled later. This trace-creation is effectively a measurement-by-environment. It’s possible to imagine that the brain evolved to leverage brief coherence for useful computation, but ultimately always “records the result” in classical form for durability. This way, even if the quantum state is fleeting, its effects are cumulative and lasting in the classical domain (learning, decision outcomes, etc.).
In summary, decoherence is the process that likely limits and shapes quantum consciousness. The Self Lens model must incorporate it by acknowledging that most large-scale brain states will appear classical due to interaction with the body and environment. The open question is whether any pockets of isolation or novel mechanisms (like those posited by Orch-OR or quantum error correction strategies) allow some coherence to survive long enough to be functionally relevant. An intellectually honest stance is that as of now, evidence for such long-lived coherence in the brain is scant, but from a theoretical angle we can chart out what would be needed and what it would imply. Decoherence theory provides a quantitative framework for this: one can calculate coherence lengths and times, and design experiments to test for them. The coming together of consciousness studies with quantum decoherence research could yield experiments (for example, interference tests with neural activity or careful entanglement probes) that either detect a faint quantum order in neural processes or further reinforce the classical viewpoint. Either outcome teaches us something – if quantum coherence is found, the Self Lens model gains empirical support; if it’s not, the model might need to retreat to being a purely metaphorical or mathematical analogy rather than a literal physical description.
Entropy, Information, and Entropic Gradients in the Brain
Considering consciousness as a physical system allows us to apply thermodynamic and information-theoretic principles to it. Entropy, a central concept in both thermodynamics and information theory, plays a key role in understanding the flow and transformation of information in a quantum brain model. In thermodynamic terms, entropy measures the disorder or randomness of a system, and isolated systems tend to evolve toward states of higher entropy (the Second Law). In information terms, entropy measures uncertainty or missing information about the state. A conscious brain is an open system (it exchanges energy and information with its environment), but we can still talk about entropy within the brain and entropy of the brain+environment.
One intriguing idea is to look at entropic gradients as drivers of cognitive processes. An entropic gradient means a difference in entropy between two places or states, which can create a kind of “flow” as the system moves toward equilibrium. The brain, being highly structured and low-entropy compared to a random jumble of matter, constantly works against increasing entropy by using energy (glucose metabolism powers neuron firing and maintenance of order). In doing so, it maintains negentropy (negative entropy) to keep memories intact, signals coherent, etc. From a broad perspective, one could say the brain sustains an entropy gradient between itself and the surroundings – exporting heat and waste (higher entropy) to keep its internal states more ordered, similar to how a refrigerator pumps out heat to keep its interior cool. This is not unique to the brain; all life does this. But in terms of consciousness, some theorists like Karl Friston have suggested that brains also minimize “entropy” or “free energy” in an information sense – the brain strives to reduce surprise (unexpected uncertainty) by updating its model of the world. This is essentially navigating entropic gradients in a space of probability distributions: moving from high uncertainty to lower uncertainty as we learn or perceive.
In a quantum context, we can discuss entanglement entropy. If we partition the brain’s quantum state into two parts (say, two neural assemblies or subsystems A and B), the entanglement entropy is a measure of how much uncertainty one part has about the other – or how inseparable they are. High entanglement entropy means the subsystems share a lot of quantum information and cannot be described independently (they are strongly correlated). This resonates with the idea of integration in consciousness (Tononi’s Integrated Information Theory, IIT, is all about how much a system’s parts act as a unified whole). While IIT is usually formulated classically, one could imagine a quantum version where entanglement plays the role of connecting parts. If consciousness corresponds to a system state that is highly entangled across many components, then the entanglement entropy between any partition (e.g., left vs right hemisphere, or visual cortex vs rest of brain) might be very high when one is conscious and alert, and lower when unconscious (where parts of the brain might behave more independently). Some researchers have indeed speculated about entropy of brain activity correlating with consciousness level – for example, the “entropic brain hypothesis” in neuroscience suggests that certain altered states (like psychedelic states) have increased entropy in neural dynamics as measured by signal diversity, which correlates with more fluid, less constrained cognition. In quantum terms, that could correspond to a brain state exploring a wider superposition (higher entropy means a broader mixture of states accessible). By contrast, focused attention or deep sleep might be lower entropy states (the brain settles into a more limited repertoire of states, perhaps a more narrow wavefunction or one dominated by a few basis states).
Now, entropic gradients within the brain’s state space could drive transitions. For instance, if a certain configuration of the brain has much lower entropy (more ordered firing pattern) than alternatives, achieving that configuration from a higher-entropy state might require work (energy expenditure) or some driven process. One might view attention as a process that locally reduces entropy: focusing on a specific task or stimulus tends to organize neural activity (many neurons lock into a coordinated pattern, extraneous activity is suppressed), which is a reduction of disorder. This would be moving down an entropic gradient – akin to a Maxwell’s demon, the brain selectively allows certain information in and keeps noise out to reduce uncertainty about the object of attention. Of course, the Second Law isn’t violated because this ordering produces heat and consumes energy, increasing entropy elsewhere (in blood flow and heat dissipation). The Self Lens model, being fundamentally about consciousness shaping reality, might interpret this as the self actively carving out low-entropy pockets of order (meaningful information structures) in the surrounding chaos. The math of entropic gradients could be incorporated via entropy terms in the Lagrangian or Hamiltonian of the model. For example, one could include a term that penalizes high entropy in the conscious subspace or that represents the coupling to a heat bath (environment) with a certain temperature. Minimization of free energy (energy minus temperature times entropy) could emerge as a principle for steady conscious states, echoing the free-energy principle of brain function (which is an information-theoretic reformulation of maintaining order against noise).
From the standpoint of quantum information theory, one can quantify how much information the brain-as-quantum-system holds and processes. A pure quantum state has entropy zero (since it’s completely specified), but if we are ignorant of some aspects or if the state is mixed, we can talk about von Neumann entropy ()S(\rho) = -\mathrm{Tr}(\rho \ln \rho)() for the brain’s density matrix ρ. As the brain decoheres, ()S(\rho)() increases (loss of information from the system to environment). We might speculate that conscious processing tries to minimize the increase of entropy by perhaps isolating certain processes or promptly error-correcting (if such mechanisms exist). Alternatively, one could imagine consciousness leveraging entropy production for creativity: random perturbations (effectively drawing from an entropy reservoir) might help generate novel ideas or explore state space, akin to simulated annealing algorithms that add noise to escape local minima. The balance between order and chaos, so often discussed in complex systems theory of the brain, here can be framed as a balance between maintaining low entropy (to preserve information and stable identity) and allowing some entropy (to enable flexibility and exploration). The term “entropic gradient” implies movement, so we might think of the brain climbing or descending entropy slopes as needed – perhaps descending (reducing entropy) when focusing or consolidating memory (making things more definite), and ascending (increasing entropy) when brainstorming or in REM sleep where brain activity becomes more random and exploratory.
Another relevant measure is mutual information between the brain and environment. A conscious agent typically has high mutual information with its surroundings (it acquires information, thereby reducing its uncertainty about the world). In quantum terms, mutual information includes classical correlations and quantum entanglement between brain and environment. The process of perception can be seen as the brain entangling with external states (hence gaining information). Once decohered, that entanglement yields classical information that the brain “knows.” The entropy reduction in the brain (lower uncertainty after learning something) corresponds to an entropy increase in the environment (the environment lost some unknown-ness, or effectively the entropy was displaced). Of course, overall entropy (brain + environment) likely increases because the measurement process has many irreversible aspects (e.g., photons being absorbed, neural signals dissipating energy). So the second law holds globally, but locally the brain can harvest information – a neat trick living systems excel at.
In sum, applying entropy and information concepts to quantum consciousness provides a bridge to statistical physics and complex systems. It encourages questions like: Is consciousness a state of low entropy (high information) compared to unconscious states? Does the brain operate near a critical point where entropy is delicately balanced with order (some have suggested brains perch at the edge of chaos, maximizing computational power)? Are there identifiable entropy flows when one becomes aware of a stimulus (i.e., a measurable reduction in uncertainty about that stimulus mirrored by changes in brain signal entropy)? The Self Lens model, by being a physical model, can incorporate these ideas quantitatively. For example, one could simulate a simplified quantum neural network and track its von Neumann entropy or entanglement entropy as it processes inputs, checking if conscious-like states correspond to particular entropy profiles. This brings us naturally to the broader view of system-level modeling.
System-Level Modeling and Emergent Complexity
Modeling consciousness as a quantum system inevitably means grappling with complex systems dynamics. The brain is complex on many scales: molecular, cellular, network, whole-organ. A true system-level model must integrate these scales or at least capture the essence of their interactions. Tools from physics and complex systems science – such as network theory, chaos theory, and statistical mechanics – become invaluable in understanding how a coherent conscious mind could emerge from a multitude of components.
One approach the Self Lens model takes is drawing analogies to quantum field theory. Instead of treating consciousness as just a single wavefunction in an abstract space, we might consider it as a field permeating the brain (and perhaps beyond). In the formal outline, the model introduced a “Higgs-like field” φ(x,t) representing a field of potential for growth, and gauge fields A_μ(x,t) for external influences. While these names are analogical, the intent is to use the powerful Lagrangian/Hamiltonian formalisms of field theory to describe interactions both within the individual (internal dynamics) and with the environment (external forces). The Lagrangian given in the outline included terms L_consciousness, L_interaction, L_external, mirroring how a particle in a field would have a free part and interaction parts. By constructing such a Lagrangian, one can in principle derive equations of motion (using the Euler-Lagrange equations or the Schrödinger equation if we go the Hamiltonian route) that describe how the state |Ψ(t)⟩ evolves over time under various influences. This is a top-down physical modeling strategy: assume consciousness is something like a particle or field described by a law (Lagrangian), then see what the solutions look like and whether they resemble known cognitive phenomena. For instance, the free Hamiltonian Ĥ_0 in L_consciousness could encode the natural tendencies of the mind (perhaps oscillatory dynamics corresponding to brain rhythms), whereas interaction terms might encode how identity, intention, and awareness operators influence each other (e.g., a term coupling the identity operator with the intention operator might represent that a strong sense of self modulates one’s goals).
From the complex systems viewpoint, the brain is a network of neurons (and glial cells, etc.), each quite complex in its own right. A quantum model could, at least conceptually, assign quantum states to components of this network. For example, individual neurons might be modeled as having a quantum state that encompasses various microstates (some have even proposed each tubulin dimer in microtubules as a quantum two-state system or “qubit”, leading to an enormous number of qubits per neuron). These quantum elements would interact (entangle) with each other when neurons connect or share signals. The entire network’s state could exhibit properties like entanglement spreading along network connections (somewhat analogous to how excitation spreads in classical neural nets, but with quantum correlations piggybacking). The mathematical tool of graph theory and networks could be used to analyze the structure of these connections, while quantum many-body physics would describe the state over that graph. This starts to look like a kind of quantum neural network, a concept that exists in quantum computing literature (quantum neural nets are studied as models that combine features of neural networks and quantum circuits). If one could simulate a quantum neural network with, say, dozens of simple “quantum neurons,” one could test how different network topologies affect coherence and entanglement, and whether the network shows any collective behavior reminiscent of consciousness (integration, dynamical stability, etc.). There is even a notion of criticality in such networks – perhaps a conscious network operates near a phase transition where it’s maximally sensitive and has long-range correlations. In classical brain models, criticality (between order and disorder) has been suggested to optimize information processing. A quantum critical brain might likewise sit at a knife-edge where it’s not completely ordered (which would freeze thought) but not totally disordered (which would be noise); instead, it’s in a complex intermediate phase with long-range entanglements and rich dynamics.
Another complex-systems concept is chaos. Chaotic systems have extreme sensitivity to initial conditions and can generate fractal, self-similar patterns. The brain shows chaotic dynamics in certain regimes, which is thought to contribute to its flexibility. The Self Lens outline even mentions chaos and fractals, noting that small changes in initial conditions (like slight shifts in intention or identity) could lead to very different growth paths, and that behavioral patterns might be fractal. In a quantum model, chaos can exist too (quantum chaos deals with quantum systems whose classical analogs are chaotic). A quantum mind could exhibit quantum chaos such that its state evolution is highly sensitive to tiny quantum fluctuations. This might underlie the unpredictability and individuality of thought processes – even if one could set up the same initial state twice (which is practically impossible due to quantum uncertainty), the slightest unobservable difference could amplify to a hugely different thought after some time. This is essentially a quantum butterfly effect in mental state space. It puts fundamental limits on predictability: no matter how well we map the brain, if consciousness has chaotic quantum dynamics, we may never predict a person’s exact thought trajectory beyond a short time window because of exponential divergence caused by hidden microscopic variables. Importantly, chaos and complexity can create structured patterns spontaneously. The brain might exploit this by shaping the chaotic attractors through learning such that they correspond to useful functional states (like having an attractor for the concept of “self” that the mind tends to return to, providing continuity of personality).
Fractals and self-similarity might appear in the patterns of brain activity across scales. Some studies have found fractal-like temporal fluctuations in neural signals. If consciousness is scale-invariant in some sense, one might see similar patterns at the micro-scale (neural microstates) and macro-scale (EEG patterns). In a quantum picture, self-similarity could tie into how states entangle: a small subsystem could entangle in a way that “echoes” the entanglement structure of larger systems (perhaps analogous to renormalization group ideas where you see repeating structure at different scales).
Bringing these ideas together, the Self Lens model at a system level envisions a multi-layered quantum system: from fundamental quantum degrees of freedom (fields, particles, spins) up through molecules, neurons, circuits, brain regions, and the whole self, each layer following physical laws but also giving rise to emergent laws at higher levels. The “lens” metaphor implies the self might be focusing or selecting among these dynamics. In physics terms, one could imagine the self as a collective variable (like the center of mass in mechanics, or an order parameter in phase transitions) that summarizes the state of the whole system in a meaningful way. For example, maybe the degree of global phase coherence could serve as an order parameter for consciousness (with a high value when the system is unified and conscious, dropping when the system fragments in sleep or under anesthesia). This would be akin to magnetization in a ferromagnet: above the critical temperature (high entropy, disordered spins), magnetization is zero (no global order); below it (low entropy, ordered spins) magnetization is nonzero. If we apply that analogy, perhaps the waking conscious brain is an ordered phase (not spatially ordered like spins, but phase-aligned in some multidimensional sense) whereas the unconscious brain is a disordered phase. The transition might be smooth or abrupt depending on conditions (some authors have indeed modeled anesthetic-induced unconsciousness as a phase transition in brain dynamics).
The computational aspect of such a model is also worth noting. If consciousness is running on quantum hardware (the brain), what kind of computations can it do? There’s speculation that certain cognitive feats (like insight problems or very fast recognition) might be easier if quantum processes were involved, though no clear example demands quantum computation yet. Nonetheless, if we think in terms of complexity classes, a quantum brain could potentially tackle problems in the complexity class BQP (quantum polynomial time) which includes some tasks believed to be intractable for classical brains (assuming the brain as a classical computer). This is highly conjectural, but it’s tantalizing to think that evolution might have stumbled on ways to exploit quantum effects for survival advantages (speed or efficiency of information processing). Tools from quantum computing theory might then inform neuroscience: for instance, quantum error correction algorithms might be analogous to neural mechanisms to preserve information in noisy conditions; quantum algorithms like Grover’s search (quadratically speeding search in unsorted databases) might inspire hypotheses about memory retrieval processes if the brain had something analogously superposed.
Finally, intellectual honesty demands we also consider that a lot of these physics-heavy constructs may serve as metaphors or approximations rather than literal descriptions. The Hilbert space model and associated physics concepts (coherence, measurement, entanglement, etc.) provide a rigorous language and perhaps a direction to unify mind and matter under one framework. Whether consciousness actually exploits quantum coherence or whether a similar formalism could just be an effective description of very complex classical computations (like a useful fiction) is an open question. The Self Lens model asserts a strong form of the idea – that consciousness is truly quantum – and pushes this hypothesis to see how far it can go. By doing so, it spurs testable questions and detailed theoretical work, such as: What specific physical structures could carry the quantum degrees of freedom (e.g., spins in neural proteins)? What are the time and length scales of any protected coherence in those structures, and do they line up with known cognitive timing (e.g., 100 Hz gamma oscillations or 40 Hz integration cycles)? Can we detect quantum entanglement between separated brain regions (perhaps via nonlocal correlations in neural signals that can’t be explained classically)? Can quantum-inspired models (like using Hilbert space formalisms) predict psychological phenomena better than classical models? These are difficult but fascinating lines of inquiry.
In conclusion of this chapter, by mapping consciousness onto the language of quantum physics, the Self Lens model opens a playground of rigorous concepts – from Hilbert spaces and Hamiltonians to entropy and entanglement – applied to one of science’s greatest mysteries. This continuation has looked into the structural and computational implications of the model: a conscious mind as a state in an immense Hilbert space, possibly maintaining coherent superpositions; a self that acts like an observer, performing measurements (sometimes even weakly) to collapse possibilities into lived reality; an ongoing battle against decoherence to retain quantum information just long enough to matter; entropic and information-theoretic principles guiding the flow of conscious states; and the hope of capturing the emergent complexity of the brain with tools akin to those that have succeeded in physics. While much of this remains theoretical, it sets the stage for a deeper scientific engagement with the Self Lens hypothesis. By being precise in our terms and unabashedly mathematical in our approach, we ensure that the discussion stays grounded. The next steps would involve translating these ideas into concrete models or experiments, forging a path where consciousness research and quantum physics inform each other. In doing so, we continue to sharpen the Self Lens – focusing it on objective, quantifiable aspects of conscious experience – until perhaps one day, the clarity of insight matches that which physics has achieved for the material world.
The Self Lens: A Quantum Model of Consciousness
The Quantum Nature of Mind
What if consciousness itself operates according to quantum principles? The Self Lens model proposes exactly this: that our minds function not merely as classical information processors but as quantum systems, with all the strange and powerful properties that entails. This isn't simply a metaphor. Rather, it suggests that the mathematics describing quantum systems—superposition, entanglement, measurement, and collapse—might literally describe how consciousness works.
This perspective invites us to see thoughts, feelings, and perceptions as quantum states existing in an abstract mathematical space. When you experience indecision or hold contradictory emotions simultaneously, perhaps you're experiencing a genuine quantum superposition. When you feel deeply connected to another person, maybe that's a form of quantum entanglement between minds. And when you focus your attention, collapsing possibilities into a single experience, perhaps you're performing something akin to quantum measurement.
The implications are profound. If consciousness truly operates according to quantum principles, we gain a new framework for understanding its most puzzling aspects—from the binding problem (how diverse sensory inputs become unified experience) to the nature of choice and even the possibility of non-local connections between minds. The Self Lens model offers a bold hypothesis: that the deepest truths of physics and the most intimate facts of experience might share the same underlying structure.
Consciousness in Hilbert Space
To formalize this quantum view of consciousness, we need a mathematical framework. In quantum mechanics, this framework is Hilbert space—an abstract mathematical space where quantum states "live." The Self Lens model proposes that each possible state of consciousness corresponds to a vector in an enormously high-dimensional Hilbert space.
Think of this space as containing all possible experiences you could have. Each point in this space represents a complete state of consciousness at a given moment—including all sensations, thoughts, emotions, and self-awareness. Your conscious experience from moment to moment traces a path through this vast space of possibilities.
Mathematically, we can represent your conscious state at any time as a vector |Ψ(t)⟩. This state can be expressed as a combination of more basic states:
|Ψ(t)⟩ = ∑ᵢ cᵢ(t) |i⟩
Here, the |i⟩ represent fundamental experiential states (perhaps corresponding to basic perceptions or mental elements), while the coefficients cᵢ(t) determine how much each basic state contributes to your overall experience at time t. Because these coefficients can be complex numbers, they carry both magnitude (how strongly that component is present) and phase (how it relates temporally to other components).
This mathematical structure allows us to define operators that act on conscious states. For example, we might define an "identity operator" that measures aspects of self-concept, an "awareness operator" that measures levels of mindfulness, or an "intention operator" that captures goal-directed focus. These operators would have eigenstates—states with definite values of identity, awareness, or intention—and measuring these aspects of consciousness would collapse the state into one of these eigenstates.
While speculative, this framework provides a rigorous language for discussing consciousness. It suggests that before we "observe" or focus on a particular thought or feeling, our mind exists in a superposition of possibilities. The act of attention collapses this superposition into a definite experience—much as measuring a quantum particle collapses its wavefunction into a definite position.
The Power of Superposition
One of quantum mechanics' most counterintuitive features is superposition—the ability of quantum systems to exist in multiple states simultaneously until measured. Applied to consciousness, this suggests our minds might hold contradictory states in genuine superposition rather than merely switching rapidly between them.
Consider a moment of indecision. Classical psychology might say you're rapidly alternating between options or holding competing neural patterns. The quantum view suggests something more profound: your mind literally exists in a superposition of both choices until the moment of decision "collapses" the state.
We might represent this as:
|Ψ⟩ = α |Option A⟩ + β |Option B⟩
The coefficients α and β determine the probability of each choice when you finally decide. But before that moment, you're not simply uncertain—your mind actually contains both possibilities simultaneously.
This perspective offers a new way to understand ambiguity in thought. Creative insights often emerge from holding multiple perspectives simultaneously. Artists and scientists frequently describe breakthroughs as seeing connections between seemingly contradictory ideas. In quantum terms, this could represent maintaining a coherent superposition long enough for novel interference patterns to emerge—patterns that wouldn't appear if the mind collapsed too quickly into a single perspective.
The same applies to emotional complexity. We often experience mixed feelings that aren't simply rapid alternations between emotions but genuine blends. Love can contain elements of joy and fear; nostalgia mixes happiness and sadness. These might be true superpositions of emotional states, with complex phase relationships determining how they combine and interfere.
This quantum view also suggests why certain mental states feel richer than others. A mind in coherent superposition contains more information and possibility than one collapsed into a single definite state. The creative, open mind maintains quantum coherence longer, exploring the interference patterns between possibilities before collapsing into conclusions.
Phase Coherence and Mental Unity
In quantum systems, phase relationships between components determine how they interfere. When components have aligned phases, they interfere constructively, amplifying each other. When out of phase, they interfere destructively, canceling each other out. This phase coherence might be crucial for understanding how consciousness maintains unity.
The binding problem in neuroscience asks how diverse neural processes combine into unified experience. In classical terms, this is often attributed to synchronized neural firing. The quantum perspective suggests something deeper: perhaps consciousness maintains phase coherence across its components, creating a genuinely unified quantum state rather than merely synchronized classical processes.
When you perceive an object, multiple sensory qualities (color, shape, texture) combine into a single percept. In quantum terms, these qualities might exist in coherent superposition, their phase relationships determining how they unify. Similarly, when thinking about a complex concept, multiple aspects cohere into a single understanding through phase relationships that allow constructive interference.
This coherence might extend to the sense of self. The feeling of being a unified "I" despite having multiple aspects (roles, memories, traits) could reflect quantum coherence across these elements. When this coherence weakens—as perhaps happens in certain dissociative states or mental disorders—the unified self fragments.
Maintaining such coherence in the warm, wet environment of the brain presents a significant challenge. Quantum systems typically decohere rapidly when interacting with their environment. For quantum effects to matter in consciousness, the brain would need mechanisms to preserve coherence long enough to be functionally relevant.
Some theorists have proposed that certain neural structures—like microtubules within neurons—might provide protected environments where quantum coherence could persist. These structures might shield quantum states from environmental interference through mechanisms like quantum error correction or ordered water molecules that screen thermal fluctuations.
While highly speculative, such mechanisms would allow the brain to harness quantum coherence for consciousness. If successful, they would enable a form of information processing fundamentally different from classical computation—one where multiple possibilities exist simultaneously and interfere according to their phase relationships.
Attention as Quantum Measurement
In quantum mechanics, measurement causes a superposed state to collapse into a definite outcome. The Self Lens model proposes that attention plays an analogous role in consciousness—collapsing the mind's quantum superposition into specific experiences.
Before you focus on something, your consciousness might contain numerous potential experiences in superposition. When you direct attention to one aspect, this superposition collapses, bringing that aspect into clear awareness while other possibilities fade. This explains why attention feels like a selective process—it literally selects one reality from many possibilities.
For example, when entering a room, your mind initially holds multiple potential perceptions in superposition. As you focus on a particular object, your perceptual state collapses around it, bringing it into sharp awareness while other elements recede into the background. Similarly, when contemplating a decision, focusing attention collapses the superposition of options into a specific choice.
This perspective raises a profound question: who or what performs this measurement? In physics, this is known as the measurement problem—if everything is quantum, what causes collapse? Some interpretations suggest consciousness itself might be the ultimate observer that collapses quantum states.
The Self Lens model takes an intriguing position: consciousness is both the quantum system being observed and the observer doing the observing. When you become aware of your own thoughts, you're performing a kind of self-measurement, collapsing your own quantum state.
This self-referential quality aligns with contemplative traditions that speak of "witness consciousness"—a fundamental awareness that observes all mental phenomena without itself being an object. In quantum terms, this might be the final observer in the chain, the measuring agency that doesn't require another observer.
The model also accommodates the possibility of partial or weak measurements. Sometimes we sense something vaguely without fully focusing on it—an intuition, a peripheral awareness, a subliminal perception. These might correspond to weak quantum measurements that extract some information without fully collapsing the state.
When you have a hunch about something without clear conceptual understanding, you might be performing a weak measurement on your mental state—gaining partial information while preserving some quantum coherence. This allows the state to continue evolving and potentially yield insights that wouldn't emerge from immediate collapse into definite concepts.
Decoherence and Mental Rigidity
If consciousness has quantum properties, why don't we constantly experience bizarre quantum effects? The answer lies in decoherence—the process by which quantum systems lose their quantum properties through interaction with their environment.
When a quantum system interacts with its surroundings, its delicate phase relationships spread into the environment, effectively becoming classical. The system no longer exhibits quantum behavior like superposition or interference. Instead, it behaves as if it's in a definite classical state, even though technically it's entangled with its environment.
In the brain, decoherence would occur extremely rapidly due to constant interactions with the warm, wet neural environment. Calculations suggest quantum states in neurons should decohere in trillionths of a second—far faster than neural firing times (milliseconds). This poses a significant challenge for quantum consciousness theories.
Proponents of quantum consciousness have proposed various mechanisms to mitigate decoherence. Some suggest specialized cellular structures might shield quantum processes from environmental interference. Others propose that quantum effects might be orchestrated to occur before decoherence destroys them, perhaps through quantum gravity mechanisms that cause objective reduction of the wavefunction.
Regardless of the specific mechanism, decoherence helps explain why consciousness typically appears classical rather than quantum. Most of our mental processes decohere rapidly, yielding definite classical experiences. This is actually necessary for stable experience—if our perceptions remained in perpetual superposition, we couldn't function in a world that demands definite actions.
Decoherence also offers insight into mental rigidity. Over time, repeated patterns of thought and perception become "measured" by the environment, collapsing into habitual classical states. Your sense of identity, emotional responses, and conceptual frameworks gradually decohere from quantum potentiality into classical certainty.
A child's mind might explore wildly, maintaining many superpositions of imagination and identity. But as environmental feedback repeatedly "measures" certain states (through social reinforcement, physical constraints, etc.), these possibilities collapse into more definite patterns. The adult mind becomes more predictable, with fewer quantum possibilities and more classical certainty.
This perspective suggests that mental flexibility requires protecting quantum coherence—creating conditions where the mind can explore superpositions before premature collapse. Practices like meditation might serve this function by temporarily isolating consciousness from environmental measurement, allowing quantum-like exploration of mental states.
Weak Measurements and Intuition
Between full quantum coherence and complete classical collapse lies an intermediate realm of weak measurement—where a system is partially observed, extracting some information without destroying all quantum properties. This concept offers insight into subtle aspects of consciousness like intuition, peripheral awareness, and subliminal perception.
In quantum physics, a weak measurement gently probes a system, gaining limited information while minimally disturbing its state. After a weak measurement, the system remains largely in superposition, though slightly altered. The measurement yields a "weak value" that provides a hint about the system without forcing it into a definite state.
Applied to consciousness, weak measurement might explain how we can sense something without fully focusing on it. When you have a vague intuition about a problem's solution, you might be weakly measuring your mental state—extracting partial information without collapsing the full superposition of possibilities. This allows continued quantum processing until a stronger measurement (focused attention) yields a definite insight.
Similarly, peripheral awareness might involve weak measurements of environmental stimuli. You notice movement in your visual periphery without fully attending to it—a weak measurement that provides some information while preserving your focus elsewhere. And subliminal perception could represent extremely weak measurements that influence your mental state without reaching the threshold for conscious awareness.
Weak measurements yield interesting properties in quantum physics. Weak values can sometimes lie outside the range of eigenvalues for the measured observable, reflecting interference between pre- and post-selected states. Similarly, intuitions can sometimes yield exaggerated or seemingly impossible insights that later prove valuable—as if accessing information beyond normal measurement bounds.
This framework suggests that consciousness isn't simply binary (aware or unaware) but exists along a spectrum of measurement strength. From the vaguest subliminal influence to the sharpest focused attention, consciousness performs measurements of varying intensity on its own states and the environment.
The ability to modulate measurement strength might be crucial for optimal cognition. Too much strong measurement (constant focused attention) would collapse all quantum potentiality, preventing creative connections. Too little measurement would leave the mind in an incoherent superposition without definite content. Balancing these extremes—knowing when to measure weakly (remain open) and when to measure strongly (focus decisively)—could be a key aspect of mental development.
Entropy and Information in Quantum Consciousness
Viewing consciousness through a quantum lens allows us to apply concepts from thermodynamics and information theory. Entropy—a measure of disorder or uncertainty—becomes particularly relevant for understanding how consciousness processes information.
In thermodynamic terms, the brain maintains low entropy (high order) by consuming energy and exporting waste heat. This allows it to sustain complex, organized neural patterns rather than dissolving into random noise. In information terms, low entropy means high certainty or knowledge—the brain reduces uncertainty about its environment through perception and learning.
A quantum perspective adds new dimensions to this picture. Quantum states can contain superpositions that represent multiple possibilities simultaneously. The entropy of such states reflects not just classical uncertainty (which option is true?) but quantum uncertainty (how these possibilities interfere and coexist).
When consciousness collapses a superposition through measurement, it reduces quantum entropy—selecting one definite outcome from many possibilities. This collapse creates information by transforming potential into actual. Before measurement, the state contains many interfering possibilities; after measurement, it contains one definite reality plus a record of that measurement.
This process might underlie how consciousness creates meaningful experience from raw sensory data. The quantum state initially contains superposed interpretations of ambiguous stimuli. Through measurement (attention), consciousness collapses this superposition into a definite perception, reducing entropy locally while increasing it elsewhere (as the discarded possibilities dissipate into the environment).
We can also consider entanglement entropy—a measure of how inseparable different parts of a quantum system are. If consciousness involves quantum entanglement across neural systems, the entanglement entropy between brain regions might correlate with the unity of experience. High entanglement entropy would indicate that different aspects of consciousness (visual, auditory, conceptual) aren't independent but form an integrated whole.
This aligns with theories like Integrated Information Theory, which proposes that consciousness corresponds to a system's capacity to unify information. In quantum terms, this unity might manifest as entanglement across neural subsystems, creating a state that cannot be decomposed into separate parts.
Entropy gradients—differences in entropy between states or regions—might drive conscious processes. Attention could be seen as moving down an entropy gradient, creating local order (focused awareness) at the cost of increased entropy elsewhere (suppressed distractions). Creative insight might involve finding low-entropy patterns (coherent ideas) within high-entropy search spaces (seemingly unrelated concepts).
The balance between order and disorder appears crucial for consciousness. Too much order (low entropy) yields rigid, predictable states with little capacity for novel information. Too much disorder (high entropy) yields chaotic states without stable patterns. Consciousness might operate at a critical point between these extremes—ordered enough to maintain coherent experience but disordered enough to adapt and create.
This critical balance could explain why consciousness seems poised between determinism and randomness. It's neither completely predictable nor completely chaotic, but exists in a complex intermediate regime where quantum effects might provide just enough indeterminism to enable creativity and choice while maintaining sufficient order for coherent thought.
Emergent Complexity in the Quantum Brain
The brain's complexity spans multiple scales—from molecules to cells to networks to the whole organ. A quantum model of consciousness must address how quantum effects at microscopic scales could influence macroscopic experience. This requires understanding how complexity emerges across these scales.
One approach treats consciousness as a quantum field permeating the brain rather than a single wavefunction. Like electromagnetic fields in physics, this consciousness field would have dynamics governed by field equations, potentially including terms for internal processes and external influences. Such a field could support wave-like phenomena, with interference patterns corresponding to thoughts or perceptions.
At the network level, we might envision neurons as nodes in a quantum network, with quantum states entangling across synaptic connections. This would create a vastly complex quantum system where information flows not just through classical signaling but through quantum correlations. The topology of this network—which nodes connect to which—would shape how quantum states evolve and entangle.
This quantum neural network might operate near a critical point between order and chaos. In classical complex systems, criticality maximizes information processing capacity—the system is neither too rigid (unable to respond to new inputs) nor too chaotic (unable to maintain stable patterns). A quantum critical brain might similarly balance coherence and decoherence, maintaining quantum effects just long enough to be computationally useful before collapsing into classical outputs.
The concept of chaos—extreme sensitivity to initial conditions—takes on new meaning in quantum systems. Quantum chaos could amplify tiny quantum fluctuations into macroscopic differences in thought patterns, creating a fundamental unpredictability in mental processes. Even if we could perfectly map the brain's state, quantum uncertainty would limit our ability to predict future thoughts beyond a short time horizon.
Yet this chaos isn't pure randomness. It can generate complex, structured patterns—strange attractors in the state space of consciousness. Learning might shape these attractors, creating stable patterns that the mind naturally gravitates toward (like familiar concepts or emotional responses) while still allowing for variation and creativity within those patterns.
Self-similarity across scales—a hallmark of complex systems—might manifest in how quantum states organize in the brain. Patterns of entanglement might repeat at different levels, from molecular interactions to neural assemblies to whole-brain states. This fractal-like structure could enable information to flow seamlessly across scales, unifying microscopic quantum processes with macroscopic conscious experience.
From a computational perspective, a quantum brain could potentially perform operations impossible for classical systems. Quantum parallelism allows simultaneous evaluation of many possibilities; quantum tunneling enables transitions between states that would be classically forbidden; quantum interference can amplify certain pathways while suppressing others. These quantum computational advantages might underlie cognitive feats that seem difficult to explain classically, like creative insight or rapid pattern recognition.
Whether consciousness literally exploits quantum computation or whether quantum formalism simply provides a useful mathematical description remains an open question. The Self Lens model takes the stronger position—that consciousness genuinely operates according to quantum principles—while acknowledging the need for empirical validation.
This leads to testable questions: What physical structures in the brain could support quantum coherence? What timescales would these quantum processes operate on, and do they align with known cognitive timescales? Can we detect quantum correlations between brain regions that classical models cannot explain? Addressing these questions requires bridging quantum physics and neuroscience in novel ways.
Philosophical Implications
If consciousness truly operates according to quantum principles, the philosophical implications are profound. The model offers fresh perspectives on longstanding questions about mind, matter, free will, and the nature of reality.
The "hard problem" of consciousness—how physical processes give rise to subjective experience—takes on new dimensions. If consciousness is fundamentally quantum, the gap between physical description and subjective experience might narrow. Quantum states inherently involve superposition, interference, and non-locality—properties that seem more aligned with the holistic, unified nature of experience than classical physical states.
This perspective suggests consciousness might not emerge from classical brain processes but from quantum properties that were always present in matter. The brain would not create consciousness ex nihilo but would organize and amplify quantum properties into the rich experience we know. Consciousness would be intrinsic to reality rather than an epiphenomenal byproduct.
The mind-body relationship would be reconceived as a dual-aspect monism: mind and matter as complementary manifestations of an underlying quantum reality. The physical brain, observed externally, appears as neurons and synapses; the same system, experienced internally, manifests as consciousness. This avoids both dualism (mind and matter as separate substances) and reductive materialism (mind as merely brain function).
Free will gains new footing in this framework. Classical physics is deterministic—given initial conditions, future states follow necessarily. Quantum physics introduces genuine indeterminism—outcomes are not fixed until measurement. If consciousness interacts with quantum indeterminacy, perhaps through attention (measurement), it could influence outcomes within the bounds of quantum probability.
This wouldn't be unlimited freedom—quantum probabilities still constrain possible outcomes—but neither would it be pure determinism or randomness. It would be a middle path where consciousness participates in determining which potential becomes actual, within the framework of physical law.
The nature of self takes on quantum dimensions as well. Rather than a fixed entity, the self might be more like a pattern of quantum coherence—a relatively stable but dynamic organization of quantum information. This aligns with contemplative traditions that describe the self as both empty (without fixed essence) and luminous (actively aware).
The boundaries between self and world become more fluid in this picture. If consciousness involves quantum entanglement, the strict separation between observer and observed dissolves. Your conscious state might be subtly entangled with others and with the environment, creating connections that transcend classical boundaries.
This suggests a more participatory view of reality—consciousness doesn't merely observe a pre-existing world but participates in determining which potential reality actualizes. Through attention and intention (quantum measurement and state preparation), consciousness helps shape the world it experiences.
These philosophical implications remain speculative, but they illustrate how a quantum model of consciousness could transform our understanding of ourselves and reality. By bridging the languages of physics and experience, the Self Lens model offers a framework where science and subjectivity might finally converge.
Practical Applications and Testable Hypotheses
While theoretical, the quantum consciousness framework suggests practical applications and empirical tests. If consciousness operates according to quantum principles, this should manifest in observable phenomena.
One approach involves testing for quantum signatures in neural activity. Quantum systems exhibit distinctive patterns like interference, entanglement, and contextuality that classical systems cannot replicate. Sophisticated neuroimaging might detect quantum correlations between brain regions that violate classical bounds, suggesting genuine quantum processes.
Another avenue explores cognitive phenomena that resist classical explanation. Quantum cognition research already applies quantum probability to decision-making, finding that human choices sometimes violate classical probability laws in ways that quantum models predict. Further experiments could test whether other cognitive processes—like creativity, insight, or perception—show quantum-like properties.
The model also suggests practical applications for mental development. If consciousness involves quantum coherence, practices that enhance coherence might improve mental function. Meditation, for example, might work partly by reducing environmental "measurement" of the mind, allowing quantum states to evolve more freely before collapse.
Similarly, creativity might benefit from techniques that delay premature collapse of mental superpositions. Brainstorming methods that postpone criticism, incubation periods that allow unconscious processing, or environments that reduce external pressure might all preserve quantum-like exploration of possibilities.
For clinical applications, mental disorders could be reconceptualized in quantum terms. Depression might involve getting trapped in certain quantum attractors, unable to explore alternative states. Anxiety might reflect excessive measurement—constantly collapsing possibilities into worst-case scenarios rather than maintaining healthy superposition of outcomes. Treatments could aim to restore quantum flexibility to rigid mental patterns.
Educational approaches informed by quantum consciousness would emphasize both focused attention (effective measurement) and open awareness (maintaining coherent superpositions). Students would learn when to collapse possibilities into definite knowledge and when to preserve ambiguity for creative exploration.
Technology might eventually interface with quantum aspects of consciousness. Future brain-computer interfaces could potentially detect and respond to quantum signatures in neural activity. Quantum computers might better simulate or complement human thought if consciousness indeed operates on quantum principles.
These applications remain speculative until we better understand if and how quantum effects manifest in consciousness. The path forward requires collaboration between quantum physicists, neuroscientists, psychologists, and philosophers to design experiments that could detect quantum signatures in the mind.
Such experiments might include testing for quantum coherence in neural structures, looking for non-classical correlations in brain activity, or designing psychological tests that distinguish quantum from classical information processing. While challenging, these investigations could potentially transform our understanding of mind and open new frontiers in mental health, education, and technology.
The Path Forward: Unifying Physics and Experience
The quantum model of consciousness represents an ambitious attempt to bridge physics and subjective experience—two domains often considered irreconcilable. By applying the mathematics of quantum theory to consciousness, the Self Lens model suggests these domains might share fundamental principles.
This unification isn't merely theoretical—it has practical implications for how we understand ourselves and reality. If consciousness operates according to quantum principles, our subjective experience isn't separate from the physical world but intimately connected to its deepest nature. The mathematics describing quantum particles would also describe our thoughts and feelings, revealing a profound unity underlying apparent diversity.
This perspective invites a more participatory view of science. Rather than seeing consciousness as merely observing an independent reality, it suggests consciousness actively participates in reality's unfolding. Through attention and intention (quantum measurement and state preparation), we help determine which potentials actualize from the quantum field of possibilities.
For science itself, this implies that first-person experience deserves equal standing with third-person observation. A complete science would include both objective measurements and subjective reports, recognizing that consciousness is both the object of study and the means by which we study anything. This doesn't diminish scientific rigor but expands its scope to include the very awareness through which science happens.
The path forward requires intellectual humility. The quantum model remains speculative, and many questions await empirical investigation. Does the brain actually maintain quantum coherence long enough for it to matter functionally? How exactly would quantum effects scale up from microscopic to macroscopic levels? What specific neural structures might support quantum processes?
These questions demand collaboration across disciplines—quantum physics, neuroscience, psychology, philosophy, and contemplative traditions. Each field offers crucial insights: physics provides mathematical rigor, neuroscience grounds the model in biology, psychology connects it to behavior and experience, philosophy addresses conceptual foundations, and contemplative traditions offer first-person methods for investigating consciousness.
This collaborative approach might yield a science of consciousness that honors both objective and subjective aspects of reality. Rather than reducing mind to matter or elevating mind above matter, it would recognize mind and matter as complementary manifestations of an underlying quantum reality—neither identical nor separate, but intimately connected.
The ultimate value of the quantum consciousness framework may lie not in whether it's literally true in all details, but in how it transforms our understanding of ourselves. By suggesting that consciousness participates in reality at its most fundamental level, it offers a vision where we are neither passive observers nor separate souls, but active participants in the universe's unfolding—quantum beings whose awareness helps shape the very reality we experience.
This vision doesn't diminish the mystery of consciousness but reframes it. The question becomes not how dead matter produces consciousness, but how the inherently quantum nature of reality manifests as the rich cohesion of conscious experience. In this light, consciousness isn't an anomaly requiring explanation but an expression of nature's fundamental character—as integral to reality as space, time, or energy.
The Self Lens model thus invites us to see ourselves anew: not as classical machines accidentally housing awareness, but as quantum systems whose very nature includes consciousness. Our thoughts, feelings, choices, and connections might all reflect the quantum principles that underlie reality itself. In this view, to understand consciousness fully would be to understand the universe from the inside—to recognize that the awareness reading these words is not separate from the quantum fabric of existence but an expression of its deepest nature.