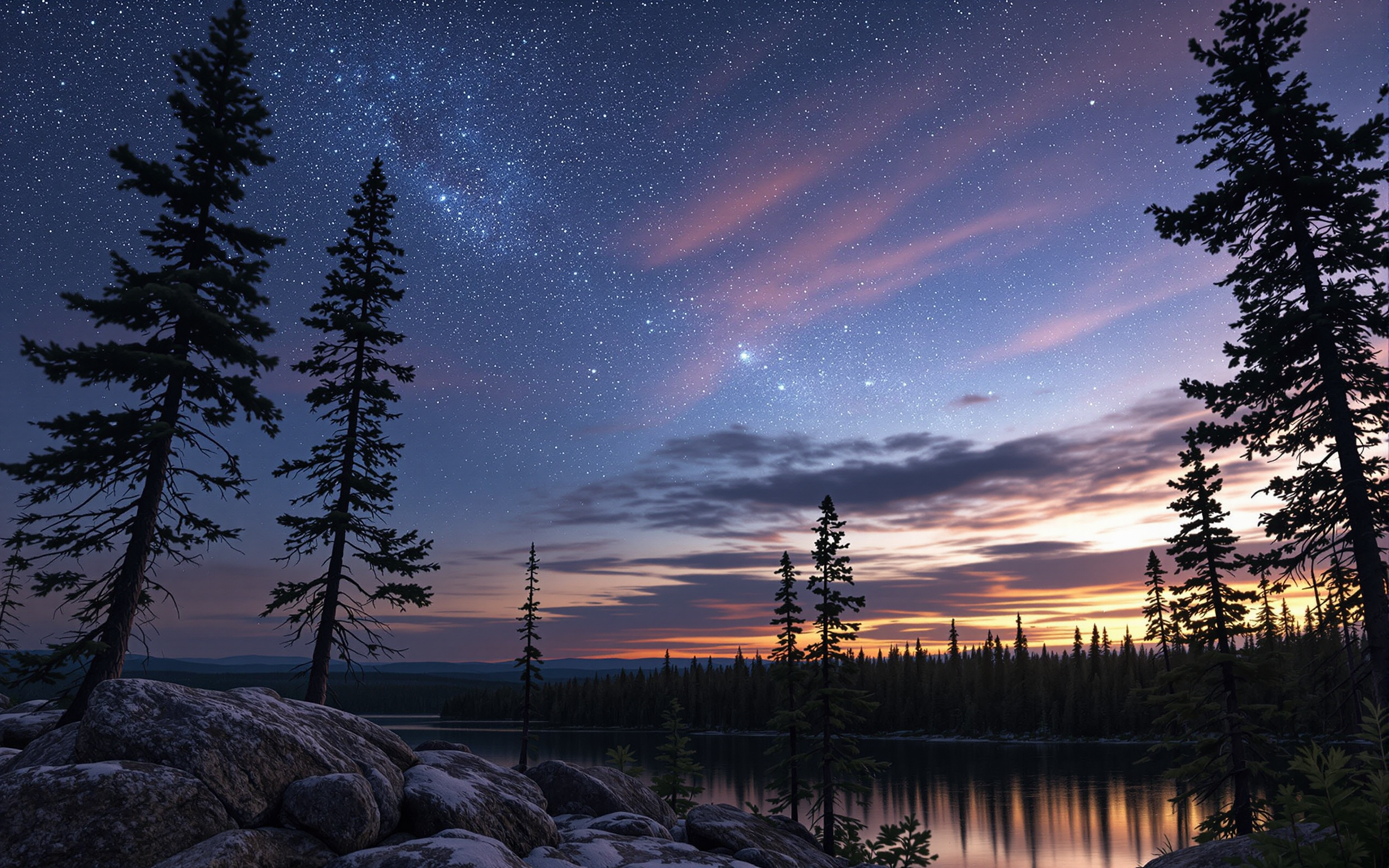
ToE Seed - Chapter 4
Chapter 4a – The Machine and the Muse
Complexity and Emergence
Complexity and emergence – the very phrase evokes a sense of something rich and dynamic unfolding from the simplest of beginnings. In this chapter, Complexity Rising: The Machine and the Muse, we trace a path through the expanding landscape of complexity – from the first faint glimmers of order in a seemingly chaotic young cosmos to the intricate, self-reflective patterns of human thought. Throughout this exploration, we strive to unify scientific insight with philosophical wonder, staying true to the Poia Theory vision. The goal is to bring clarity to how complexity blossoms and how truly novel properties emerge at higher levels of organization, all while preserving a sense of awe. Each section peels back another layer of this story, revealing how the universe, like a masterful storyteller, unfolds new chapters of structure and meaning from the building blocks of chaos.
Emergence as Self-Defining Complexity
Emergence is the profound mystery of how genuine novelty arises in nature. How does life spring from mere chemistry? How does mind arise from mere matter? How can a flock of birds wheel and turn in unison with a grace that none of the individual birds possesses alone? These phenomena are all examples of emergent properties – features of a system that are more than just the sum of its parts. Traditionally, emergence describes the surprising appearance of something new and unexpected when many simpler elements interact. For example, the wetness of water is an emergent trait not found in isolated hydrogen or oxygen molecules; only when those molecules unify into H₂O does the quality of wetness appear. Likewise, consciousness seems to arise from networks of neurons firing in a brain, even though a single neuron by itself is not conscious. Such examples illustrate the classic idea of emergence and never fail to astonish us – they show that when parts come together, the collective can exhibit behaviors and qualities that none of the parts had in isolation.
But Poia Theory invites us to look deeper. Emergence, in the Poia perspective, is not just a byproduct or an accidental outcome – it is an active process by which complexity turns back on itself and becomes self-referential. In poetic terms, this is complexity waking up. At a critical threshold of organization, a system seems to gain a life of its own: it begins to define itself rather than being defined only by its constituent pieces. What does this mean? Consider that as interactions compound, feedback loops form, and patterns reinforce themselves, the system can start to influence its own behavior. The emergent entity establishes its own identity and, in a sense, charts its own destiny within the possibilities allowed by its components. This is emergence as self-defining complexity – the point at which a pattern gains the ability to refer to itself, to maintain its own integrity, and to act in novel ways that are not directly traceable to one simple part. It’s a tipping point where something qualitatively new enters existence: a shift from a mere collection to a coordinated whole that holds together on its own terms.
From Novelty to Self-Determination: Rethinking Emergence
To appreciate this redefined idea of emergence, let’s begin with the traditional viewpoint and then expand it. Emergence has long been understood as the appearance of new properties or behaviors that are “greater than the sum of the parts.” This classical notion gives us the basic vocabulary – those surprising leaps like wetness emerging from molecules or a simple set of electrical components giving rise to the complex operations of a computer. Such emergent phenomena surprise us because nothing in the parts themselves seems to predict the rich behavior of the whole. Philosophers and scientists from Aristotle (who noted that a whole can be more than the sum of its parts) to modern complexity theorists have marveled at this principle. We see it in chemistry (novel compounds having properties distinct from the elements that compose them), in biology (metabolism emerging from a mix of enzymes and substrates), and in consciousness studies (subjective experience arising from neural activity). Each example reinforces the intuition that something more is happening when simple entities come together in just the right way.
Poia Theory builds on this foundation and pushes it further. Instead of viewing emergence as a kind of “magic” that inexplicably appears when things get complicated, Poia asks us to think of emergence as nature’s way of achieving self-determination through complexity. When does a heap of parts truly become a whole in its own right? The answer, according to Poia, is: when the interactions between parts loop around such that the system begins to reference and regulate itself. Imagine a simple pattern growing more and more complex — like a long sentence being written one word at a time. At first, the sentence may just be a list of words (parts). But at some point, it might turn into a meaningful statement about itself. In a similar way, a complex system can reach a point where it’s not just a sum of parts, but is actually talking to itself. It actively maintains a certain structure and behavior, almost as if it has an inner voice or internal guidance system.
This is a dramatic shift in perspective: emergence is not merely about novelty, it’s about the birth of a new agency. The emergent whole starts to govern its parts, at least in part, rather than being passively governed by them. A classic illustration is the emergence of mind from matter. At a low level, neurons obey electrochemical laws, but when arranged in a vastly complex network, there comes a point where “mind” appears — and that mind can in turn direct the neurons (for instance, you can consciously decide to take a deep breath, and your neurons will oblige). In Poia terms, complexity begets autonomy. The more complex a system becomes, the more it can influence its own state and behavior. So we rethink emergence as the process by which increasing complexity yields self-determination – the capacity of a system to act on its own behalf.
The Turning Point of Complexity: When Systems Define Themselves
What does it look like when complexity “wakes up” and a system defines itself? To answer this, we need to examine what changes inside a system as it grows in complexity. One key change is the development of internal feedback loops and regulatory mechanisms. As a system gains more components and interactions, it often develops ways to monitor and adjust itself. For example, consider a simple thermostat regulating a heating system. It has a feedback loop: if the temperature drops below a set point, the thermostat senses it and turns the heat on, thereby controlling its own environment. Now, a thermostat is a very rudimentary example of self-regulation, but it hints at something important: a complex system can contain loops that link its outputs back into its inputs, allowing it to respond to changes and maintain stability.
As complexity increases further, these feedback loops multiply and intertwine. The system starts preserving its own boundaries and identity. A living cell is a perfect illustration: it is composed of many molecules, but it maintains a membrane that clearly delineates “inside” from “outside.” If something perturbs the cell’s internal environment, the cell can react (pumping out excess ions, for instance, to keep conditions just right). The cell makes “decisions” in a loose sense – not conscious decisions, but automatic responses that keep it alive. At a certain point, the collection of molecules is no longer just a collection; it’s a unit that defends its integrity and continues its own existence.
This moment – when a system becomes sufficiently complex that it maintains itself and adapts on its own – is the turning point of complexity. It’s as if we have been climbing a spiral of increasing complexity, and suddenly the spiral closes into a loop, forming a circle: the system becomes self-contained. The image of a spiral that eventually loops back onto itself is a useful metaphor here. As complexity grows (imagine tracing upward along a spiral), there comes a moment when the system’s organization curves inward and closes, creating a new whole. At that moment, the system is more than just an open-ended spiral of increasing detail – it’s a circle, a coherent entity that refers to itself and sustains itself.
Let’s highlight the key features that typically mark this transition to self-defining complexity:
- Responsive Sense-and-Act: The system can sense changes in its environment and respond in a way that benefits the whole. For instance, a simple organism can detect food or danger and react accordingly; even a bacterium will swim toward nutrients and away from harmful substances.
- Boundary and Identity: The system establishes a boundary that distinguishes “me” from “not me.” By forming this boundary, it effectively names itself as a thing apart. A cell’s membrane, or an organism’s skin, or even the psychological sense of self in an animal’s mind, are examples of this defining boundary. Within that boundary, the components cooperate to uphold the identity of the whole.
- Internal Regulation: The system manages its internal conditions actively. Through feedback loops (like the thermostat example writ large), it keeps itself in a viable state. Your body, for instance, regulates temperature, pH, and energy supply with exquisite balance; this homeostasis is crucial for any autonomous life-form.
- Adaptive Goal Orientation: The system isn’t just reacting passively; it has internal goals or preferred states that it strives to maintain. A primitive cell “wants” (in a biochemical sense) to survive and replicate. An animal seeks food, shelter, reproduction – goals encoded by evolution. Once goals exist, the system can show agency: it can behave in ways that preferentially fulfill those goals, even if it means altering its environment or its own behavior.
When a system shows all these features, we recognize it as having a degree of autonomy. The parts alone don’t have this; it emerges from their organized interaction. In essence, at this turning point, the system becomes an agent of its own change. It no longer simply follows the dictates of initial conditions and external forces – it now also follows an internal drive.
(Imagine a diagram here: a rising curve or spiral representing growing complexity, which then folds into a closed loop labeled “selfhood” at a certain threshold. This visually depicts the point at which increasing complexity yields a self-sustaining, self-directing order.)
Mechanisms of Emergence: How the New Springs Forth
Understanding that emergence is a kind of awakening of complexity, we might ask: How does this process actually happen? What are the mechanisms in play that allow something fundamentally new to spring forth from simpler interactions? Poia Theory looks at both scientific principles and metaphorical insights to illuminate a few key mechanisms behind emergence:
- Complexity as a Spectrum: Emergence is not typically a single magical leap – it’s more of a continuum. Systems move from simplicity to complexity gradually, and the onset of novelty is often a gradual dawn rather than an abrupt switch. Think of how the sky lightens imperceptibly before sunrise. In the same way, as we add more components or interactions to a system, new properties can begin to glimmer even before they fully form. There’s no precise single moment where non-emergent becomes emergent; instead, simple systems shade into complex systems. For example, consider the subtle steps from a single cell to a colony of cells that communicate, and onward to a complex organism with specialized organs. At what point does “new behavior” emerge? It’s hard to pin down – novelty unfolds progressively. This spectrum view reminds us that every big emergent jump (like life or mind) likely had precursors and intermediate forms rather than appearing out of nowhere.
- Feedback Loops and Self-Regulation: Feedback is the beating heart of emergence. Positive feedback loops can amplify small quirks or fluctuations into new stable structures. Negative feedback loops can dampen disturbances and maintain stability. Together, these create a dynamic balance that allows complexity to organize itself. For instance, a few neurons firing accidentally in sync might trigger a larger network of neurons to also synchronize (positive feedback), igniting a new pattern of brain activity such as a thought or memory. On the other hand, your body uses negative feedback to keep vital signs like blood sugar and temperature within safe limits, preventing runaway chaos internally. These mechanisms show how a system can take on a life of its own: through feedback, a system’s past state influences its future state in a loop, enabling it to self-correct or self-amplify as needed. In an ecosystem, predator and prey populations regulate each other’s numbers (negative feedback leading to balance), while a slight advantage of one species can cascade into that species dominating an area (positive feedback leading to a new ecological order). Feedback loops enable a system to braid itself into a cohesive whole that can endure and adapt.
- Boundary Creation: One hallmark of emergent complexity is the drawing of a boundary – a clear delineation between the system and the rest of the world. By creating a boundary, the system essentially says “This is me, and that is outside.” This act is profound because it allows the inside to become a world of its own. A vivid example is the formation of the first protocell in the primordial ocean: imagine fatty molecules spontaneously assembling into a tiny sphere, a membrane enclosing a pocket of chemicals. All of a sudden, there’s an “inside” and an “outside” – the first cell is like a tiny soap bubble carrying its own internal chemistry. That boundary was the birth of an independent life-form, however simple. On a completely different level, consider a child in early development learning to say “I” for the first time. In that moment, the child’s mind is drawing a psychological boundary between self and other, carving out the concept of an individual identity. In both cases, something new emerges when a boundary is drawn: the system names itself and becomes a protagonist in its own story. By setting itself apart, it can begin to cultivate its own unique internal order and guide its own evolution.
- Hierarchy and Downward Causation: Emergent systems often form layers of organization – a hierarchy where simple parts form a complex whole, which can then become part of an even larger system, and so on. We see this nested hierarchy everywhere: atoms join to make molecules, molecules assemble into cells, cells build tissues, tissues form organs, organs compose organisms, and organisms create societies. At each level, the whole has properties the parts alone didn’t have. Crucially, once a higher level emerges, it can influence the lower levels – this is called downward causation. To illustrate, imagine a dance performance: individual dancers (parts) move and interact, and out of their motions an overall pattern or formation (the whole) takes shape on stage. Once the formation exists, it in turn influences the dancers – each dancer might adjust their steps to maintain the formation, following the timing and spacing dictated by the group pattern. In the same way, your mind (the whole) can affect the behavior of your neurons (the parts), or a social trend (whole) can affect how individual people (parts) behave. There is a two-way street: bottom-up causation (parts creating the whole) and top-down causation (the emergent whole guiding the parts). This interplay knits the system together across scales. One could visualize this as a series of concentric circles or a pyramid of levels, with arrows pointing upward from the base (indicating parts building up to wholes) and arrows pointing downward from the peak (indicating wholes regulating parts). This would illustrate the mutual influence between each layer of complexity.
Each of these mechanisms contributes to the alchemy of emergence. They show that emergence isn’t just a mystical notion but can be discussed in terms of concrete processes: continuous accumulation of complexity, feedback loops, boundary formation, and multi-level organization. These are like the ingredients and recipes by which nature conjures new forms of order.
Emergence Everywhere: Patterns Across Scales
Abstract principles are helpful, but emergence truly comes alive when we see it in action across different scales and domains. The incredible thing about emergent complexity is that it recurs throughout the universe, from physics and chemistry all the way to biology, mind, and society. It’s like a universal theme that plays out in various keys and tempos. Let’s look at a few vivid examples that demonstrate how the same emergent magic transpires in different contexts:
- Physical Systems – Order from Chaos: Even in the non-living world, we witness order spontaneously arising out of chaos. A classic example is the formation of convection cells in a heated fluid. Heat a thin layer of liquid from below and, beyond a certain point, random jiggling of molecules will organize into a beautiful honeycomb array of hexagonal cells (known as Bénard cells) circulating in unison. What’s happened here? There is no leader molecule or central command, yet a symmetrical pattern appears across millions of molecules – an emergent structure. Another example: when certain chemicals are mixed together, they don’t just react and then stop; they oscillate in color and concentration (as in the Belousov-Zhabotinsky reaction), creating repeating waves of chemical activity like a rhythmic heartbeat. These dissipative structures (so-called because they dissipate energy to maintain their form) hint at how life might have gotten started. Nature seems to find pockets of stability and order if energy flows through a system in the right way. We can imagine the early Earth’s oceans as a canvas of such spontaneous pattern formation, precursors to the first living cells.
- Biological Life – Autopoiesis (“Life Lights Up”): Life itself is perhaps the most awe-inspiring emergent phenomenon we know. Consider a single living cell – a microscopic, self-contained world. Inside the cell, countless chemical reactions are happening every second, yet the cell doesn’t fall apart into chaos. It actively keeps itself going: repairing its parts, expelling waste, pulling in nutrients, and replicating when the time is right. Biologists Maturana and Varela coined the term autopoiesis (Greek for “self-making”) for this remarkable process. A cell is a prime example of autopoiesis – it continuously creates and re-creates itself. We can draw an analogy: think of a cell as a tiny city. It has walls (the cell membrane) protecting an interior bustling with factories (organelles like ribosomes and mitochondria), a power plant (energy-producing mechanisms), a library of instructions (DNA in the nucleus), and a transportation network (molecular motors and pathways). Yet there is no mayor or central planner; the cell’s order emerges from the interactions of all these components following simple biochemical rules. Life “lit up” on Earth when chemistry took this emergent leap to become biology – when molecules learned not just to form patterns, but to sustain those patterns and make copies of them. From that first cell, over billions of years, life’s complexity skyrocketed. Single cells joined together as multicellular organisms, trading a solitary existence for the advantages of cooperation. In those early multicellular colonies, we see a new emergent whole – an organism – that is greater than any single cell, with cells now specializing (some became muscle cells, some nerve cells, some skin cells, etc.) to support the collective life of one being. This too was an emergent revolution: the rise of organismal life from the teamwork of cells.
- Mind and Consciousness – The Universe Aware of Itself: Climbing further up the ladder of complexity, we arrive at consciousness – an emergent property of certain biological systems (brains) that still baffles science in many ways. Somehow, the coordinated electrochemical dance of neurons in the brain gives rise to the vivid theater of the mind: sensations, thoughts, feelings, a sense of self. In the emergent view, consciousness is what happens when information flows and feedback loops in the brain become sufficiently complex that the system can model itself. Your brain has billions of neurons making trillions of connections, and out of that unfathomably intricate network arises you – the experience of being an aware entity. What’s more, this emergent consciousness then feeds back into the system that generated it: you can consciously choose to focus your attention, which in turn changes which neurons fire and how strongly. In Poia Theory, consciousness is not seen as an accidental byproduct, but as an integral aspect of reality that plays an active role. The universe, through conscious beings, becomes aware of itself and can even begin to shape itself. When we gaze up at the stars and ponder our place, it is literally stardust (our bodies are made of atoms forged in ancient stars) pondering the stars. A collection of particles has organized itself to the point where it can think, feel, and seek meaning. This is emergence at its most poignant: the cosmos giving birth to observers who can appreciate and influence the cosmos. It’s as if the “machine” of mindless matter has produced a “muse” – the spark of awareness and creativity – encapsulated in each conscious life.
- Social Systems – Collective Intelligence: Emergence doesn’t stop at individual organisms. When individuals come together, new higher-level entities can emerge: societies, cultures, economies – these have their own patterns and behaviors beyond the individuals that make them up. A human society, for example, develops norms, languages, and institutions that guide people’s lives but cannot be reduced to any single person’s actions. Consider a language: no single individual invented English or Chinese deliberately; rather, countless people interacting over generations caused a complex, rule-governed language to emerge and evolve. Yet once the language exists, it profoundly shapes how each individual thinks and communicates (another case of downward causation). Or consider an ant colony: each ant follows relatively simple rules and has no real “idea” of the colony’s overall plan, but collectively, the colony functions as a cohesive unit that can accomplish remarkable things like farming fungi, building intricate nests, and mounting organized defense – tasks far beyond the scope of a lone ant. It’s as though the colony itself is a higher-level organism, with the ants acting as its cells. In human economies, millions of people buying and selling create emergent phenomena like markets and prices; those market forces then, in turn, influence individual decisions about what to buy or sell. In all these examples, there is an intelligence of the swarm or the group that arises from the interactions of lesser intelligences. The emergent entity – be it a corporation, a city, or a culture – has properties (like cultural values or a “market mood”) that don’t reside in any single person.
(Throughout these examples, we’re invited to feel a sense of wonder at the underlying unity of this idea. One could imagine a series of illustrations here: four panels showing a simple physical pattern (like convection cells in a liquid), a living cell, a brain, and a bustling city – each one depicting emergence at a higher scale. This visual progression drives home how the motif of emergence threads through reality at all levels.)
Implications: A New Lens on Reality
Exploring emergence as self-defining complexity gives us a powerful new lens on reality. It bridges a long-standing divide in how we understand the world. On one side, reductionism teaches that to understand a complex system, we should break it down into its parts and study those parts; on the other side, holism insists that some truths only reveal themselves at the level of the whole. Emergence shows that both perspectives are crucial: the parts do matter, but so do the novel qualities of the whole. A cell is explainable in terms of chemistry up to a point, but “life” as experienced by that cell involves emergent processes like self-maintenance that we wouldn’t know about just from a list of molecular reactions. Reality, it turns out, is layered. We live in a hierarchy of levels, each built upon the ones below, yet each introducing something new – atoms to molecules, molecules to cells, cells to organisms, organisms to ecosystems, ecosystems to societies. We might think of it as a great harmonious ensemble, like a choir where each vocal part is different yet together they create a song. Each level “sings” its own melody, but together these levels stay in harmony, influencing one another and creating the rich complexity we observe in the universe.
This viewpoint has deep implications for many debates in science and philosophy. Take the age-old question of mind and matter: Is consciousness “nothing but” neurons firing? Emergence suggests that while neurons are necessary for the mind, the conscious mind as a whole has qualities (like self-awareness, intentionality, maybe even free will) that are real and not found at the level of single neurons. In other words, consciousness could be an emergent property that deserves to be understood on its own terms, not dismissed as an illusion or epiphenomenon. Similarly, when we look at society, one might ask: is a society just a collection of individuals, or is there a real collective dynamic that has to be acknowledged? The emergent perspective leans toward the latter as well – yes, individuals matter, but when we come together, new dynamics (culture, collective consciousness, a “group mind”) genuinely exist and can exert causal power.
There is also an emotional and perhaps spiritual undertone to this realization. If the universe has a built-in tendency to create new wholes, to foster emergence, then we are part of an ongoing creative process. Complexity is not just a quirk of some systems; it is the way the cosmos has consistently expressed itself since the beginning of time – by forming ever more intricate forms, eventually including us. We can find a sense of meaning in recognizing that we belong to this grand process of emergence. In a way, understanding emergence encourages us to appreciate complexity wherever we find it: from the delicate balance of an ecosystem to the unfathomable complexity of our own relationships and societies. All these are instances of nature’s creativity at work – the universe, through trial and error, spinning chaos into order, discovering what novel things it can become.
Finally, this sets a hopeful and expansive mood as we proceed. The story of emergence is profoundly optimistic: it tells us that from simple beginnings, ever greater complexity and capability can arise. Life emerged from rocks and water; mind emerged from life; perhaps higher forms of consciousness and social organization can emerge from where we are now. Poia Theory, with its unifying vision, sees this trajectory as meaningful – possibly even hinting that purpose and value (things so important to us humans) might themselves be emergent properties of the complex web of life and mind. With this lens, we go forward ready to explore more specific patterns and principles of complexity, with the understanding that at every level, something truly new can emerge and change the game.
From Simplicity to Complexity: Patterns in Evolution
If the concept of emergence explains how new qualities appear, the history of the universe offers the grand stage on which this drama has played out. In this section, we trace the path from primordial simplicity to the astonishing complexity around us today. Think of it as the universe’s own epic tale – a progression from an almost featureless beginning to a world teeming with structure, life, and mind. At the dawn of time, the cosmos was nearly uniform: energy and a few basic particles spread out with little organization. Yet over billions of years, structure crystallized out of chaos. Particles clumped into atoms, atoms bonded into molecules, molecules assembled into stars and galaxies; on some worlds, chemicals became living cells, and those cells eventually evolved into beings capable of thought. In short, the cosmic story arcs from simple unity toward rich multiplicity. Poia Theory emphasizes that this rise in complexity is not a random accident but perhaps an inherent direction in nature – a kind of subtle pull toward order (sometimes called syntropy in the Poia lexicon). Rather than seeing the universe as only running down into disorder (as entropy alone would predict), Poia encourages us to also see the counter-trend: pockets where complexity increases, suggesting the cosmos had a potential for order and complexity from the very beginning. The language of this story can be as poetic as it is scientific – for example: “From the silent darkness of the early cosmos emerged the first light; from the churning oceans of early Earth arose the first living cell; from simple single cells grew creatures that could behold the stars and wonder.” It’s an epic narrative, and it sets the stage for the idea that we, as thinking beings, are part of this vast unfolding saga of complexity.
From Unity to Multiplicity: A Cosmic Path
To ground this epic in clearer terms, let’s sketch the major chapters of complexity’s rise. The progression can be broken into distinct stages, each representing a remarkable leap where something fundamentally new appeared. We will go stage by stage – not with a dry list of eras, but with an imaginative glimpse into what each stage looked like and what new principle of organization emerged:
- Primitive Aggregation – “Chaos with Company”: In the earliest phase of the universe, things were mostly a chaotic mix. Imagine a time when all that existed were swirling particles and energy, occasionally sticking together under the influence of basic forces like gravity. In this stage, wholes are just loose collections of parts. For example, gravity pulls countless grains of cosmic dust into a planet, or billions of stars into a galaxy – but these assemblages are essentially just heaps. There’s no coordination or higher structure beyond proximity. It’s like a crowd of strangers gathered in a plaza, or grains of sand piling into a dune by the wind. They’re together, but not truly working together. The significance of this stage is that it sets the foundation: the ingredients for complexity are now not isolated, but at least collected. However, at this point a whole (say, a star cluster or a sea of chemicals) doesn’t behave much differently than the sum of its parts. It’s chaos, but now the chaos has company – parts are no longer flying solo, yet no novel pattern has emerged quite yet.
- Interactive Networks – “When Parts Start Talking”: The next leap comes when those aggregated parts begin to influence one another in systematic ways. Interactions start weaving parts into networks. Picture a simple chemical soup on the early Earth: initially, molecules might just float randomly, but eventually some molecules start to react with others, forming cycles. A molecule A might help produce molecule B, which helps produce molecule C, which in turn produces A again – voilà, a rudimentary reaction network is born. Or consider a collection of early organisms (or even simple robots) where the action of one affects the action of another, forming, say, a basic food web or a feedback chain. In this stage, relationships become as important as the parts themselves. The system’s behavior cannot be understood by looking at any single part in isolation; it’s about the connections. Think of a small town where everyone’s decisions (to trade, to cooperate, to argue) start to depend on their neighbors – a social network emerges, however primitive. Now the whole is a web of influences, not just a heap. This is when patterns and dependencies first surface: you see cause and effect looping around, creating something that has dynamics of its own. We could say the parts have started “talking” to each other, and the conversation is what gives the system a new character.
- Self-Organization – “Order for Free”: At a certain point, interactions can lead to surprising spontaneous order. This stage is characterized by patterns that form without any external guiding hand – a kind of order that arises seemingly out of nowhere, or “for free.” For instance, consider the convection cells in a heated fluid described earlier: as you heat the liquid, you pass a threshold where instead of random turbulence, you get a stable pattern of hexagonal cells with warm fluid rising in the center of each cell and cooler fluid descending at the edges. No one designs this pattern; it self-organizes because of the physics of heat flow and fluid dynamics. Similarly, in biology, think of how flocking behavior arises: each bird in a flock follows a couple of simple rules (like “don’t bump into your neighbor, and fly in the average direction of neighbors”), and suddenly you get a coordinated flock moving in unison, making mesmerizing shapes in the sky. There’s no leader bird telling the others what to do, yet the flock moves as if guided by an unseen conductor. In chemistry, certain sets of reactions will start oscillating in sync or form spiraling waves of color. This stage shows that complex patterns can emerge without a blueprint or a leader, purely from the interactions among parts. It’s “order for free” – structure born out of chaos, given the right conditions and enough interacting pieces. When a system self-organizes, it now has properties (like a stable pattern, or the ability to sustain itself for a time) that none of the individual parts possess. This is a critical step toward life and higher complexity: it’s as if nature learned how to dance without needing a choreographer, discovering stable rhythms that can spontaneously arise.
- Autopoiesis (Self-Creation) – “Life Lights Up”: Now we arrive at one of the most pivotal emergent leaps: the origin of life. At this stage, the networks of chemical reactions cross a threshold where they become self-sustaining and self-creating. The word autopoiesis means exactly that – self-creation. Imagine that in a warm little pond or deep-sea vent on the primitive Earth, certain molecules not only form networks but actually build structures that help maintain those networks. Perhaps fatty molecules form a little bubble (a membrane) around a set of reactions, as we imagined earlier with the protocell. Inside that bubble, chemical reactions go on that produce some of the very molecules (like those membrane lipids, or RNA enzymes) that keep the bubble intact and the reaction network going. Now we have a rudimentary cell – a system that actively makes and remakes itself. It takes in energy (like chemical fuel), uses it to repair its components, removes its waste, and even reproduces by splitting into two when conditions are right. In poetic terms, this is the moment when life lights up in the universe. What was just a self-organizing chemical pattern becomes a self-maintaining, and thus living, organism. The significance can’t be overstated: this is where matter gained the ability to persist and evolve on its own. The first cells were tiny and simple compared to modern cells, but they carried the spark that would ignite all future complexity. Through countless trials, those first living systems evolved and diversified, giving rise to the entire biosphere. We might picture them as the universe’s first autonomous agents – little droplets of order that could grow, split, and spread, islands of negentropy (reverse of entropy) in a sea of randomness. Every complex living creature, including us, traces back to that leap.
- Adaptive Learning – “Memory and Response”: Life didn’t stop innovating at simple self-maintenance and reproduction. As life evolved, some organisms developed ways to learn and adapt much faster than genetic evolution alone would allow. Early nervous systems appear, and even single-celled organisms can exhibit rudimentary learning (for instance, some microbes can adjust their behavior based on past stimuli). This stage of complexity is marked by the emergence of memory: information that an organism can store and use to change its behavior. Think of a primitive worm that learns to move toward a certain smell because it associates that scent with food, or even a simple immune system that “remembers” a virus it encountered before and reacts more strongly the next time. On a different scale, consider a machine learning algorithm (an artificial neural network) which isn’t alive in the biological sense, but it embodies this principle: it can adjust its internal parameters based on experience (data) to improve at a task. What all these examples share is the idea that complexity can now increase within a single lifespan, not just across generations. An animal can acquire a new trait (behavioral or cognitive) by learning, rather than waiting for random mutations and natural selection to encode that trait in DNA over eons. In the history of life, this was a huge step: the emergence of brains and adaptive behavior meant the universe now had entities that could change themselves in response to experience. It’s as if the feedback loops became so sophisticated that the system (the organism) could simulate different possibilities in its mind, remember what worked, and alter its actions accordingly. The universe started experimenting in real-time through the minds of living creatures, not just over geological ages. This acceleration opened the door to the rapid development of new behaviors and capabilities, setting the stage for culture and technology.
- Reflective Consciousness – “The Universe Becomes Self-Aware”: In the current pinnacle (as far as we know) of complexity, the process of emergence produced beings that don’t just experience the world, but experience themselves. This is reflective consciousness – having a mind that can think about its own thoughts, a self that can ponder its own existence. Humans are the prime example we know: not only do we learn and adapt, but we also have a rich inner life, self-awareness, and the ability to ask questions like “Who am I?” and “Why am I here?”. In this stage, the universe has, in a poetic sense, opened its eyes. Through us (and perhaps other conscious beings elsewhere), the matter and energy that once merely swirled blindly now has awareness. Stardust has arranged itself into brains that can consider the stars. This feedback loop is mind-bending: an emergent phenomenon (the conscious mind) is now looking back at the very process that produced it, trying to understand itself. In Poia Theory, consciousness is not seen as a random fluke but as an important and even inevitable aspect of complex organization – the cosmic mind flowering from the cosmic body, so to speak. We emphasize, too, that consciousness may feed back into evolution: with consciousness comes imagination and intention, which lead to deliberate actions that alter the environment or even the course of future evolution (for example, human technology and culture are now shaping the planet and our own development). So, in this phase, the emergent system (the conscious being) has a profound degree of self-determination: it not only reacts and learns, it reflects and chooses purposefully. This is the frontier of emergence that we are most intimately familiar with – and perhaps it’s not the end of the story. We can speculate that even higher orders of organization and awareness might emerge (for instance, a global consciousness of humanity, or advanced forms of AI, or other forms of collective intelligence beyond our current imagination). But as of now, reflective consciousness is the richest emergent phenomenon we have experienced directly.
(It’s important to note that these stages flowed into one another gradually. Nature usually doesn’t make giant leaps in an instant; it experiments at every intermediate step. For example, between simple protocells and fully modern cells, there were likely many quasi-living chemical systems; between simple learning and full self-awareness, many incremental cognitive advances. We can imagine this progression like a growing tree. The roots represent the simple beginnings in the soil of chaos. The first sprout is life emerging, then a trunk forms as life becomes more complex and robust, then branches as different life forms and intelligences diversify, and finally a bloom of self-aware consciousness at the top. The branches overlap and growth is continuous – a reminder that the categories we’ve described aren’t strict boxes, but more like landmarks on a continuous path of increasing complexity. A visual timeline or layered diagram could help encapsulate this path: from the Big Bang and the formation of particles, to early Earth’s chemistry, to single cells, multicellular life, animals with brains, and conscious humans – each stage building on the prior and blending into the next.)
Forces Guiding the Rise of Complexity
Given this grand sweep from particles to conscious minds, a question arises: what drives this whole process? Why did the universe’s complexity keep ratcheting up instead of plateauing or collapsing back into chaos? Poia Theory identifies several key principles or “forces” that seem to consistently push systems toward greater complexity and organization. These aren’t forces in the sense of new fundamental physics like gravity or electromagnetism, but more like recurring themes or factors that enable complexity to blossom. Understanding these drivers deepens our insight into why complexity happens and hints that the universe has certain habits or tendencies that favor the growth of order under the right conditions. Here are a few of the major ones:
- Flow of Energy: Complex systems are energy-hungry. To build and maintain order, there must be a constant throughput of energy. The sun powers the complexity on Earth by showering it with sunlight, which plants capture to build sugars, which then feed animals, and so on. A planet without an energy source remains simple and dead; with energy, there’s a chance for life. We can liken energy to the fuel of creation – it doesn’t guarantee complexity, but without it, complexity cannot survive. Importantly, the energy must be harnessed or utilized by the system. A lightning bolt hitting the ocean delivers energy, but it’s chaotic and often destructive; in contrast, a plant’s chlorophyll carefully captures sunlight in a controlled way, turning it into useful chemical energy. When a system can take in energy and channel it into building internal structure (like a cell building proteins, or a city using electricity to run infrastructure), it can grow in complexity. Energy flow is also what allows life to keep fighting entropy (the tendency of things to fall apart). As long as there’s a supply of usable energy, a complex system can keep repairing itself and even building greater complexity. That’s why the hotspots of complexity (like the Earth, or any life-bearing world) are places with abundant energy gradients (sunlight, chemical fuel, geothermal heat, etc.). In summary: no energy, no complexity; abundant, well-utilized energy, and complexity can flourish.
- Information and Communication: If energy is the fuel, information is the blueprint and communication is the coordination signal. Complexity increases dramatically when parts of a system can store and share information. In biological terms, DNA is a great example of information storage – it holds the instructions to build an organism, encoding complex structures in a stable, inheritable form. Neurons in a brain share information rapidly via electrical and chemical signals, allowing different regions of a brain to coordinate and create an overall mental state. In human society, language and writing are information carriers that let knowledge accumulate and spread, leading to cultural and technological complexity beyond what any isolated person could achieve. Communication — whether chemical, neural, linguistic, or digital — ties parts together into a coherent whole. A poetic way to put it is: information is the thread that weaves separate pieces into one fabric. The better a system gets at handling information, the more complex behaviors it can exhibit. Consider two early life forms: one has no genetic memory and just randomly reacts to the environment, while another has DNA (genetic information) and perhaps the ability to send chemical signals to others. The second will likely develop more sophisticated organization over time. In short, complexity thrives on knowledge (even if it’s just a bacterium’s chemical “knowledge” of where food is) and on the ability of parts to talk to each other and coordinate their actions.
- Resonance and Coherence: This is a concept especially emphasized in Poia Theory. Resonance refers to components of a system naturally synchronizing or aligning with each other through repeated interactions. When parts resonate, they effectively join forces. Think of resonance literally: if you have tuning forks of the same frequency and you strike one, the others will start humming along. In systems, when elements fall into rhythmic alignment, the system becomes more cohesive. For example, consider how fireflies in a field will sometimes all flash in unison. Initially each insect flashes on its own random timer, but as they observe each other, they adjust until a whole population is blinking in synchrony. There’s no leader firefly – it’s a resonant synchronization that emerges. What does this do? It creates a larger-scale order (a whole field of flashing lights) which might help in communication or mating. Coherence is related; it means the parts of the system aren’t jumbled or working at cross purposes, but are instead cooperating or at least complementing each other’s actions. We could imagine coherence as being like a team rowing a boat in sync – if they row out of sync, the oars clash and the boat veers erratically; in sync, the boat moves forward smoothly. In physical systems, lasers are coherent light (all the light waves in step, producing a powerful focused beam) whereas a regular light bulb emits incoherent light (waves out of step, spreading out in all directions). A coherent system can often achieve things (sustain itself, transmit power or information efficiently, etc.) that an incoherent collection can’t. Poia suggests that resonance – a kind of sympathetic vibration among parts – is a subtle but pervasive factor that knits complexity together. It is almost as if when things “get on the same wavelength,” they naturally form a higher-order unity. Whether it’s heart cells in an embryo synchronizing their contractions to produce a heartbeat, or members of a team sharing a common vision, resonance and coherence allow a smooth merging into a functioning whole. This principle has a somewhat musical quality: just as individual notes, when in harmony, create a chord, individual components in resonance create an emergent order.
- Syntropy (Pull Toward Order): Classical physics highlights entropy, the tendency of closed systems to move toward disorder. Poia Theory, inspired by some speculative ideas in modern thought, introduces the concept of syntropy – essentially the opposite of entropy. Syntropy is a hypothetical tendency towards increasing order and complexity. While entropy is like a push from the past (things break down as time moves forward), syntropy can be thought of as a pull from the future – the idea that there are attractors or future states of higher organization that systems naturally evolve toward. This is admittedly a non-standard idea (originating from thinkers like Luigi Fantappiè and expanded by others like Vannini), but it’s a fascinating one. We can frame it more intuitively: if you throw random ingredients together, entropy says they’ll mix into a uniform mush; syntropy suggests there might be pathways where those ingredients self-organize into something interesting under the right conditions (like crystals, or life, or consciousness). It’s as if nature has a built-in preference or bias not just to break things down, but also to build things up when possible. One might imagine that the future “wants” to happen in certain ways, pulling the present toward particular complex outcomes. For example, one could whimsically say the emergence of life was “bound to happen” because there was a pull toward that complexity, or that consciousness is the universe inevitably trying to know itself. While this idea of syntropy is speculative, it gives a sense of purposefulness to the evolution of complexity – that perhaps the incredible sequence from simplicity to complexity was not a complete fluke, but part of the cosmos’s deeper potential. In any case, whether one subscribes to the idea of syntropy or not, it’s clear that certain processes in nature (like natural selection, or chemical self-organization) consistently yield increased order, almost as if searching out valleys of stability or peaks of complexity amidst the randomness.
- Consciousness as a Driver: In a unique twist, Poia Theory suggests that consciousness – which we usually think of as a result of complexity – might also itself contribute to further complexity. Once consciousness emerges (even at rudimentary levels, as in animals that have simple awareness), it can become an active participant in evolution. How so? Through the choices and intentions of conscious agents. For instance, the cultural evolution of humans has far outpaced our biological evolution, because once we had language and abstract thought, we started inventing things, sharing knowledge, and reshaping our environment. Conscious beings can set goals, cooperate, compete, and innovate, all of which drive the emergence of new complex structures (like social systems, technologies, art, and science). Even in biological evolution, the presence of consciousness could feed back – e.g. animals with preferences might choose mates or environments in ways that influence which genes get passed on. Some thinkers even propose that consciousness has a role in quantum physics – the controversial idea that observers might influence the outcomes of quantum events, hinting that awareness could bias how reality unfolds at the smallest scales. Poia doesn’t claim to resolve those controversies, but it raises the intriguing point that once the universe generated observers, those observers in turn might help to shape what emerges next. It’s a way of seeing consciousness not just as a passenger on the cosmic path, but as a co-driver in the later stages. This gives us a personal connection to the story of complexity: our own minds might be part of the engine pushing complexity forward (for example, when a scientist designs a new technology, or when society collectively imagines and implements new forms of organization). In short, awareness adds a new kind of feedback loop to the mix – a reflective, purposeful feedback – potentially accelerating and guiding the evolution of complexity in directions that raw chance alone might not achieve.
These guiding forces or principles overlap and intertwine. Together they help explain why, once the ball of complexity got rolling, it tended to build momentum. They also provide a preview of topics we will dig deeper into as we continue through Complexity Rising (for instance, the idea of syntropy and the role of consciousness in reality will get more attention in later chapters). The takeaway here is that the rise of complexity is driven – it’s not a passive, unlikely miracle, but a process fueled by energy, enabled by information exchange, glued together by resonance, perhaps beckoned by syntropy, and increasingly, steered by consciousness. The universe has some reliable strategies for growing order, and it has been using them for eons.
Complexity as the Universe’s Creative Expression
Stepping back from the details, we can reflect on what this all means in a grand sense. The evolution of complexity can be seen as the universe expressing its creative potential. Out of the simple laws of physics and a seething cauldron of particles at the Big Bang, the cosmos has somehow written, over 13.8 billion years, an elaborate story – one that includes galaxies, planets, life, and mind. Each emergent layer (from atoms to molecules to cells to ecosystems to human societies) has added a new “verse” to this cosmic epic. It’s as if the universe has been gradually crafting a masterpiece of increasing complexity over cosmic time. One might imagine the cosmos as a painter continually adding to a canvas – beginning with broad strokes of simple form and then layering in ever-finer details and structures. Or we could liken it to an author writing a story, each chapter introducing new characters and twists that would have been unimaginable in the chapter before. Whatever metaphor we choose, the essence is that the universe keeps building upon prior creations, exploring its own potential by reaching for new levels of order and novelty.
There is a sense of wonder and meaning that comes from seeing things this way. It suggests that complexity is deeply woven into the fabric of reality, not just an anomaly or a fluke. We, as humans, can take both pride and solace in being part of this great sequence. We’re not just insignificant specks; in fact, we are carriers of the universe’s complexity to its current pinnacle, participants in the very process that shaped us. The Poia perspective would highlight that perhaps the universe intended (or at least naturally allowed) conscious life as a way to know itself – hence the theme of this book, The Machine and the Muse, implying that the cold machinery of physics has given rise to the inner muse of awareness and creativity.
Looking at the world through this lens could influence how we find purpose. For example, one might argue that "the point of it all" (Poia) is for complexity to rise and for consciousness to deepen. In a very real way, when we strive to learn, to create art, to build compassionate communities, we are continuing the cosmic work of emergence: we are pushing complexity (be it intellectual, emotional, or social complexity) to new heights. This gives a kind of directionality – not a rigid predetermination, but a thematic direction – to the history of the universe: the cosmos explores what it can become by creating ever more complex forms, and thereby discovers new realms of being.
As we conclude this chapter, we set the stage for the next explorations in Complexity Rising: The Machine and the Muse. Having appreciated how complexity grows and yields emergence from simple beginnings, we are ready to examine specific patterns and mathematical principles that underlie this process. In the upcoming chapters, we will dive into concepts like fractals (patterns that repeat across scales), the mathematics of self-organization, and detailed case studies of emergence in various domains (biological, social, technological). With the broad narrative in mind, these details will further enrich our understanding. For now, we carry forward a key insight: complexity is not just an accident of the universe – it is one of its crowning achievements, and an ongoing creative endeavor. We are both products and agents of this complexity, endowed with the capacity to appreciate it and perhaps to guide its future evolution.
Chapter 4b: Complexity Rising - The Emergence of Order
Introduction: The Paradox of Increasing Order
In a universe governed by the second law of thermodynamics—where systems naturally tend toward increasing disorder—something remarkable happens. Life emerges. Consciousness develops. Complex structures form and evolve. This apparent contradiction represents one of the most profound puzzles in science: how does increasing complexity and order arise in a cosmos seemingly predisposed toward entropy and chaos?
The Poia Theory addresses this paradox by examining the principles of emergence, self-organization, and complexity. These concepts reveal how simple components, following basic rules of interaction, can spontaneously generate astonishingly complex and ordered systems. From the intricate patterns of snowflakes to the vast neural networks of the human brain, nature demonstrates an inherent tendency to create order from simplicity when certain conditions are met.
This chapter explores how complexity emerges across different scales of reality—from physical systems to biological organisms to conscious minds. We'll examine the mathematical principles that govern self-organization, the role of feedback loops in creating stable complex systems, and how consciousness itself might represent an emergent property of underlying complexity. Through this exploration, we'll see how the apparent contradiction between entropy and increasing order resolves into a deeper understanding of nature's creative processes.
The emergence of complexity doesn't contradict the laws of thermodynamics but complements them. Complex systems can develop and maintain their order by drawing energy from their environment and dissipating entropy outward. This process creates local pockets of increasing organization within the larger context of universal entropy increase. Understanding this balance between order and disorder provides crucial insights into how consciousness might interact with physical reality and how the universe evolves toward greater complexity.
Emergence occurs when interactions between simple components generate properties or behaviors that couldn't be predicted from understanding the components in isolation. The whole becomes not just greater than but different from the sum of its parts. This phenomenon appears throughout nature at every scale, from quantum fields to cosmic structures.
Consider water. Individual H₂O molecules possess certain properties—a specific atomic structure, electrical charge distribution, and behavior under different conditions. Yet when trillions of these molecules interact, entirely new properties emerge: wetness, surface tension, wave formation, and crystalline structures in ice. None of these properties exist at the level of individual molecules. They emerge only through collective interaction.
This pattern repeats across reality. Individual neurons firing in simple patterns create the emergent phenomenon of consciousness. Individual birds following basic rules of separation, alignment, and cohesion create the emergent phenomenon of flocking behavior. Individual humans engaging in economic exchanges create the emergent phenomenon of markets and economies.
Emergence follows several key principles:
Nonlinearity: Small changes in initial conditions can produce disproportionately large effects in emergent systems. This nonlinearity makes emergent phenomena inherently unpredictable from reductionist analysis alone.
Self-Organization: Complex patterns form without centralized control or external direction. The order emerges from the interactions between components following simple local rules.
Boundary Conditions: Emergence requires appropriate constraints that channel interactions in productive ways. Too many constraints prevent emergence; too few lead to chaos rather than complexity.
Energy Flow: Sustained emergence requires continuous energy flow through the system. This energy maintains the far-from-equilibrium state necessary for complex organization.
These principles help explain how complexity arises spontaneously throughout nature. They suggest that the universe possesses an inherent tendency toward self-organization under the right conditions—not as a violation of entropy but as a complementary process that creates temporary, localized order within the larger context of increasing disorder.
The concept of emergence challenges purely reductionist approaches to understanding reality. While reductionism provides valuable insights by analyzing components, emergence reminds us that new properties and principles appear at each level of organization. Understanding water molecules doesn't fully explain ocean waves; understanding neurons doesn't fully explain consciousness; understanding individuals doesn't fully explain societies.
This multi-level perspective aligns with the Poia framework's integration of physical and conscious aspects of reality. Consciousness itself may represent an emergent property—not reducible to its physical components but arising from their complex interactions. This emergent view provides a middle path between strict materialism (which struggles to account for subjective experience) and dualism (which struggles to explain mind-body interaction).
Self-Organization: Order Without Design
One of the most counterintuitive aspects of complexity is how ordered patterns can emerge without any central designer or controller. Self-organization—the spontaneous formation of structure through local interactions—appears throughout nature, from crystal formation to the development of biological organisms to the functioning of economies and ecosystems.
Self-organization challenges our intuitive assumption that order requires a designer. When we see intricate patterns in nature—the hexagonal cells of a honeycomb, the spiral arrangement of seeds in a sunflower, the branching structure of rivers and trees—we might assume some central blueprint or plan guided their formation. Yet these patterns often emerge from simple components following basic rules of interaction, with no central control or predetermined design.
Several key mechanisms drive self-organization:
Positive Feedback: Small initial differences amplify over time, creating distinct patterns or structures. For example, a slight depression in sand might catch more water during rainfall, deepening the depression and eventually forming a stream bed.
Negative Feedback: Stabilizing mechanisms prevent runaway growth or collapse, maintaining the system within functional parameters. For example, predator-prey relationships help maintain population balances in ecosystems.
Autocatalysis: Processes that accelerate their own production, creating self-reinforcing cycles. For example, certain chemical reactions produce catalysts that speed up the same reaction, leading to exponential growth until resources limit further expansion.
Critical Thresholds: Many self-organizing systems exhibit phase transitions when certain parameters reach critical values. At these thresholds, the system's behavior changes qualitatively, often displaying new emergent properties.
These mechanisms operate across diverse systems. The Belousov-Zhabotinsky reaction demonstrates how simple chemical interactions can produce complex, oscillating patterns. Slime molds with no central nervous system can solve maze problems and create efficient networks. Sand dunes form regular patterns through the interaction of wind and sand particles. In each case, order emerges without design.
The mathematics of self-organization often involves nonlinear differential equations that describe how system components change over time based on their interactions. These equations frequently generate solutions showing how complex patterns can emerge from simple rules. For example, the reaction-diffusion equations developed by Alan Turing explain how leopards get their spots and zebras their stripes through the interaction of two chemicals—one that activates growth and another that inhibits it.
Self-organization provides a powerful framework for understanding how complexity arises in the universe without requiring external design. It suggests that the fundamental laws of physics and chemistry contain within them the potential for generating extraordinary complexity when energy flows through systems under appropriate boundary conditions.
This perspective has profound implications for how we understand consciousness and its relationship to physical reality. If consciousness represents an emergent property of complex neural systems, then it arises not through some external intervention but through the self-organizing principles inherent in nature itself. The human mind, with its remarkable capacities for self-awareness, abstract thought, and creative imagination, may represent the natural unfolding of complexity through self-organization rather than something imposed from outside the physical world.
Complexity Theory: The Mathematics of Emergence
Complexity theory provides mathematical tools for understanding how order emerges from simple interactions. Unlike classical physics, which excels at describing simple systems with few variables, complexity theory addresses systems with many interacting components that generate unexpected collective behaviors.
At the heart of complexity theory lies the concept of complex adaptive systems—networks of components that learn and evolve through interaction with their environment. These systems share several key characteristics:
Nonlinear Dynamics: Small causes can produce large effects, and the relationship between inputs and outputs isn't proportional. This nonlinearity creates the potential for sudden transitions, tipping points, and emergent behaviors that can't be predicted from linear extrapolation.
Sensitivity to Initial Conditions: Often called the "butterfly effect," this principle states that tiny differences in starting conditions can lead to vastly different outcomes over time. This sensitivity makes long-term prediction inherently limited, even in deterministic systems.
Attractor States: Despite their unpredictability, complex systems often gravitate toward particular patterns or states called attractors. These can be fixed points (like a pendulum coming to rest), periodic cycles (like a heartbeat), or strange attractors (like weather patterns that never exactly repeat but maintain recognizable characteristics).
Fractal Patterns: Many complex systems generate self-similar patterns that repeat at different scales. Fractals like the Mandelbrot set demonstrate how simple recursive equations can produce infinitely complex boundaries. Similar fractal patterns appear throughout nature—in coastlines, tree branches, blood vessel networks, and mountain ranges.
The mathematics of complexity often involves concepts from chaos theory, network theory, and information theory. For example, the logistic map equation—a simple formula for population growth—demonstrates how a system can transition from stable behavior to chaotic fluctuations as a single parameter increases:
xₙ₊₁ = rxₙ(1-xₙ)
Where x represents population size and r represents the growth rate. As r increases, the system moves from stable equilibrium to periodic oscillations to chaotic behavior—a pattern seen in many natural systems.
Network theory provides another mathematical framework for understanding complexity. It examines how the structure of connections between components influences system behavior. Many complex systems display "small-world" network properties—most nodes connect to nearby neighbors, but occasional long-distance connections create shortcuts across the network. This structure, found in systems from neural networks to social media, enables both local specialization and global integration.
Information theory contributes the concept of entropy as a measure of uncertainty or disorder. Complex systems often operate at the "edge of chaos"—a state between rigid order and complete randomness where information processing capacity is maximized. This balance point allows for both stability and adaptability, a crucial feature of living systems.
The mathematics of complexity reveals how simple rules can generate extraordinary diversity and order. Cellular automata—systems where cells on a grid change state based on simple rules about their neighbors—demonstrate this principle dramatically. Conway's Game of Life, a famous cellular automaton, shows how just a few simple rules can generate patterns capable of universal computation.
These mathematical insights help explain how consciousness might emerge from neural complexity. The human brain, with its approximately 86 billion neurons and 100 trillion synapses, represents one of the most complex systems known. Its self-organizing networks, operating at the edge of chaos, create the conditions for consciousness to emerge as a higher-order property of the system—not reducible to individual neurons but arising from their collective dynamics.
Complexity theory thus provides a mathematical foundation for understanding how consciousness could emerge from physical processes without being identical to them. It offers a framework that respects both the physical basis of mind and the unique properties that emerge at the level of conscious experience.
Fractals: Self-Similarity Across Scales
Fractals represent one of nature's most fascinating expressions of complexity—patterns that repeat themselves at different scales, creating infinite detail through simple recursive processes. From the branching of trees and rivers to the structure of lungs and blood vessels, fractal patterns appear throughout the natural world, revealing an underlying order that transcends specific scales.
The essential characteristic of fractals is self-similarity—the property where parts resemble the whole at different magnifications. Zoom in on a small section of a fractal, and you'll find a pattern similar to the larger structure. This self-similarity can be exact (as in mathematical fractals) or statistical (as in natural fractals like coastlines or mountains).
Mathematician Benoit Mandelbrot coined the term "fractal" and pioneered their study, recognizing that many natural structures couldn't be described using traditional Euclidean geometry. The coastline paradox illustrates this concept: the measured length of a coastline increases as the measuring unit decreases, potentially approaching infinity as the measurement scale approaches zero. This occurs because coastlines contain detail at every scale—each bay contains smaller inlets, which contain still smaller features, and so on.
The mathematics of fractals often involves iterative equations where the output of one calculation becomes the input for the next. The Mandelbrot set—perhaps the most famous mathematical fractal—emerges from the simple equation:
zₙ₊₁ = zₙ² + c
Where z and c are complex numbers. Despite this simplicity, the resulting pattern contains infinite complexity, with elaborate structures appearing at every level of magnification.
Fractals demonstrate several important principles relevant to the Poia framework:
Complexity from Simplicity: Extraordinarily complex patterns can emerge from simple rules applied recursively. This principle extends beyond mathematics to physical, biological, and social systems.
Scale Invariance: Some patterns and principles remain consistent across different scales of reality. This suggests that certain fundamental organizing principles might apply from quantum systems to cosmic structures.
Efficient Organization: Fractal structures often represent optimal solutions for certain problems. For example, the fractal branching of lungs maximizes surface area for gas exchange while minimizing the space required.
Boundary Complexity: Fractals often appear at boundaries between order and chaos, or between different states of a system. This connects to the idea that consciousness might operate at the boundary between potential and actuality.
The prevalence of fractal patterns in biological systems suggests they represent a fundamental organizing principle in life. The human body contains numerous fractal structures—the branching of nerves, blood vessels, and bronchial tubes; the folding patterns of the brain cortex; the network of specialized cells in organs. These patterns maximize functional capacity while maintaining adaptability.
Consciousness itself might exhibit fractal-like properties. The recursive nature of self-awareness—the ability to think about thinking, to be aware of being aware—creates a kind of self-similarity across levels of cognition. Similarly, the relationship between individual and collective consciousness might display fractal patterns, with similar dynamics appearing at different scales of social organization.
The fractal perspective offers a powerful metaphor for understanding the relationship between parts and wholes in complex systems. Each component contains, in some sense, information about the entire system—just as each part of a hologram contains information about the whole image. This connects to the holographic principle discussed in quantum physics and suggests how individual consciousness might access information from the broader field of awareness.
Fractals thus provide both a mathematical description of complexity and a conceptual bridge between different levels of reality. They demonstrate how simple principles can generate infinite complexity and how patterns can maintain consistency across vastly different scales—from the quantum to the cosmic, from the neural to the conscious.
Feedback Loops: The Engines of Complexity
Feedback loops—processes where outputs circle back to become inputs—serve as the primary mechanisms driving complexity in natural systems. These loops create the conditions for self-organization, adaptation, and evolution, allowing systems to maintain stability while simultaneously developing increasingly complex structures.
Two primary types of feedback operate in complex systems:
Negative Feedback stabilizes systems by counteracting changes. When a system deviates from equilibrium, negative feedback pushes it back toward balance. Your body's temperature regulation exemplifies this process: if you overheat, you sweat to cool down; if you get too cold, you shiver to generate heat. These responses counteract the initial change, maintaining your temperature within a narrow range. Negative feedback creates homeostasis—the ability of living systems to maintain internal stability despite external fluctuations.
Positive Feedback amplifies changes, pushing systems away from their current state. A small initial difference grows larger over time through reinforcement. For example, in population growth, more individuals lead to more reproduction, creating more individuals in an expanding cycle. Similarly, in financial markets, rising prices can attract more buyers, pushing prices higher still. Positive feedback creates the conditions for exponential growth, rapid change, and the emergence of new structures.
The interplay between these feedback types generates complexity. Negative feedback alone produces stability but prevents evolution; positive feedback alone leads to runaway growth or collapse. Complex adaptive systems balance these forces—using positive feedback to explore new possibilities and negative feedback to stabilize useful discoveries.
This balance appears throughout nature. In neural development, positive feedback strengthens frequently used connections while negative feedback prevents overexcitation. In ecosystems, positive feedback drives population growth while negative feedback from predation and resource limitations prevents overpopulation. In economies, positive feedback rewards successful innovations while negative feedback from competition prevents monopolies.
Feedback loops often operate across different timescales and levels of organization, creating nested cycles that regulate each other. For example, in biological systems:
-Fast feedback loops regulate moment-to-moment processes like neural firing
-Medium-speed loops control developmental processes like cell differentiation
-Slow loops guide evolutionary adaptation across generations
This multi-level regulation creates robust yet adaptable systems that can maintain stability while evolving new capabilities.
The mathematics of feedback often involves differential equations that describe how system variables change over time based on their current state. For example, the logistic growth equation models population growth with resource limitations:
dN/dt = rN(1-N/K)
Where N is population size, r is the growth rate, and K is the carrying capacity. This equation captures how positive feedback (reproduction) interacts with negative feedback (resource limitations) to create a stable equilibrium.
More complex systems require more sophisticated mathematical models, often involving multiple coupled differential equations or agent-based simulations. These models can reveal how feedback loops generate emergent behaviors that wouldn't be predicted from analyzing components in isolation.
Consciousness itself may emerge from feedback loops in neural systems. The global neuronal workspace theory suggests that consciousness arises when information from specialized brain modules enters a shared workspace where it can influence and be influenced by other processes. This creates a feedback loop where perception affects thought, thought affects attention, attention affects perception, and so on—generating the integrated experience we call consciousness.
The Poia framework extends this understanding by suggesting that consciousness not only emerges from neural feedback loops but participates in broader feedback cycles with physical reality. When consciousness observes and intends, it influences which potentials manifest from the quantum field; these manifestations then affect subsequent observations and intentions, creating a continuous feedback loop between mind and matter.
This perspective helps explain why consciousness evolved—it provides an adaptive advantage by enabling organisms to participate more effectively in reality's feedback cycles. Rather than passively responding to environmental pressures, conscious beings can anticipate, plan, and intentionally shape their circumstances, creating positive feedback loops that accelerate their development.
Understanding feedback loops thus provides crucial insights into how complexity emerges, how consciousness arises from physical processes, and how mind and matter might interact in a unified reality. These cycles of influence and response represent the engines that drive increasing complexity throughout the cosmos.
The Edge of Chaos: Where Complexity Thrives
Complex systems achieve their greatest creativity and computational power in a narrow region between rigid order and random chaos—a state known as "the edge of chaos." This boundary zone represents the sweet spot where systems maintain enough structure to preserve information but enough flexibility to evolve and adapt.
Systems operating in highly ordered states exhibit stability and predictability but lack adaptability. Like perfect crystals, they maintain their structure but cannot easily reorganize to meet new challenges. Conversely, systems in chaotic states show great flexibility but cannot maintain stable patterns long enough to build complexity. Like gases, they change constantly but cannot develop organized structures.
The edge of chaos balances these extremes. Here, systems maintain enough order to preserve useful patterns while remaining flexible enough to explore new possibilities. This balance creates the conditions for complexity to emerge and evolve.
This principle appears across diverse systems:
Cellular Automata: Computer scientist Stephen Wolfram classified cellular automata (grid-based systems following simple update rules) into four classes. Class 4 automata—those operating at the edge of chaos—demonstrate the most complex behavior, including the capacity for universal computation.
Neural Networks: Brains function most effectively when neural activity balances between under-stimulation and over-stimulation. Too little activity prevents information processing; too much creates seizure-like states where useful patterns dissolve into noise. Healthy brains maintain this critical balance.
Ecosystems: The most diverse and resilient ecosystems exist in the middle range of disturbance frequencies—not so stable that dominant species crowd out others, but not so frequently disturbed that communities cannot establish themselves.
Social Systems: Innovative organizations balance structure and flexibility, maintaining enough rules for coordination while allowing enough freedom for creativity. Too much hierarchy stifles innovation; too little prevents effective collaboration.
The mathematics of this boundary state often involves phase transitions—points where systems change their qualitative behavior as parameters cross critical thresholds. Near these transition points, systems show characteristic behaviors:
-Long-range correlations, where changes in one area influence distant regions
-Power-law distributions, where events of all sizes occur with specific frequency patterns
-Critical slowing down, where systems take increasingly long to return to equilibrium after disturbances
-Increased information processing capacity, where the system can perform more complex computations
These properties emerge when the system reaches a state of self-organized criticality—a condition where it naturally evolves toward and maintains itself at the critical point between order and chaos. The classic example is a sand pile: as sand grains drop onto the pile, it builds until it reaches a critical slope. At this point, adding a single grain can trigger avalanches of any size, from tiny shifts to massive collapses. The system maintains itself at this critical state through its own dynamics.
Consciousness may represent a phenomenon that emerges precisely at this boundary between order and chaos. The human brain maintains itself near this critical state, with neural activity showing the characteristic patterns of systems at the edge of chaos—including power-law distributions of activity and long-range correlations across brain regions. This critical state maximizes the brain's information processing capacity, allowing it to balance stability and flexibility in responding to a complex world.
The edge of chaos concept connects to the Poia framework's understanding of consciousness as the bridge between potential and actuality. The quantum field of potential represents maximum possibility but minimum actualization—like a chaotic system with infinite flexibility but no stable structure. Manifest reality represents maximum actualization but reduced possibility—like an ordered system with stable structure but limited flexibility. Consciousness operates at the boundary between these domains, selecting which potentials become actual while maintaining awareness of multiple possibilities.
This boundary position may explain consciousness's unique capabilities. By operating at the edge of chaos, consciousness can both maintain stable patterns (memories, identity, beliefs) and explore new possibilities (creativity, learning, adaptation). It can preserve information from the past while remaining open to future developments. This balance creates the conditions for consciousness to serve as an effective bridge between potential and actuality.
Understanding complex systems at the edge of chaos thus provides insights into both how consciousness emerges from physical processes and how it functions as an interface between potential and manifest reality. The same principles that generate complexity throughout nature may underlie the emergence of mind itself.
Autopoiesis: Self-Creating Systems
Autopoiesis—literally "self-creation"—describes systems that continuously generate and maintain themselves through their own processes. Introduced by biologists Humberto Maturana and Francisco Varela, this concept helps explain how living systems maintain their identity and boundaries despite constant material exchange with their environment.
An autopoietic system creates the very components that produce it. Consider a living cell: it produces enzymes that catalyze reactions that produce more enzymes, all while maintaining the cell membrane that separates this process from the environment. The cell's components constantly break down and are replaced, yet the overall organization persists. The cell creates itself continuously.
This self-creating quality distinguishes living systems from machines. A car doesn't produce its own parts or repair itself when damaged. It's an allopoietic system—created by external processes rather than by its own operations. Living systems, in contrast, maintain themselves through internal processes, achieving a form of autonomy while remaining dependent on environmental resources.
Autopoiesis involves several key characteristics:
Operational Closure: The system's processes form a closed network where each component helps produce other components. This closure creates the system's identity and distinguishes it from its environment.
Structural Coupling: Despite operational closure, the system maintains ongoing interaction with its environment, exchanging matter and energy while preserving its organization. This coupling allows adaptation without loss of identity.
Emergent Boundaries: The system creates its own boundaries that separate it from the environment. These boundaries aren't just physical barriers but dynamic processes that regulate what enters and leaves the system.
Self-Reference: The system takes its own organization as a reference point for its operations. It responds to environmental perturbations based on how they affect its internal organization rather than processing "objective" information.
These principles extend beyond individual cells to more complex living systems. Multicellular organisms, ecosystems, and even social systems can display autopoietic characteristics—continuously regenerating their components while maintaining their overall organization.
The concept of autopoiesis connects to consciousness in several ways. First, consciousness itself may represent an autopoietic process—a self-creating system of awareness that maintains its continuity while constantly changing its contents. The sense of self emerges from this continuous self-creation rather than from any fixed substance or structure.
Second, autopoiesis helps explain how consciousness relates to its physical substrate. The mind-brain relationship resembles the relationship between a living system's organization and its material components. Just as a cell's identity persists despite complete molecular turnover, consciousness maintains continuity despite changing neural states. The pattern persists while the substrate changes.
Third, autopoiesis suggests how consciousness might participate in reality creation. If consciousness operates autopoietically, it continuously creates the conditions for its own existence through its operations. By selecting which potentials to actualize from the field of possibility, consciousness shapes the reality it experiences, which in turn influences future conscious states—a self-reinforcing cycle of creation.
The mathematics of autopoiesis often involves concepts from systems theory and topology. Mathematically, an autopoietic system can be modeled as a network of processes where the output of each process serves as input for others, creating a closed loop of production. This closure doesn't mean isolation from the environment but rather organizational autonomy—the system's processes determine how it responds to environmental influences.
This mathematical perspective helps explain how consciousness could be simultaneously dependent on physical processes and irreducible to them. The mind emerges from neural activity but achieves a form of operational closure that gives it causal efficacy in its own right. Consciousness isn't separate from physical reality but represents a particular organization of physical processes that achieves self-creation and self-maintenance.
Autopoiesis thus provides a powerful framework for understanding how consciousness emerges from physical processes while maintaining its distinctive qualities. It suggests that mind isn't a substance or a property but an ongoing process of self-creation—a dynamic organization that continuously regenerates itself through its own operations while remaining coupled to the physical world.
Syntropy: The Counterbalance to Entropy
While entropy describes the universe's tendency toward increasing disorder, syntropy represents the complementary tendency toward increasing order and complexity in certain systems. First proposed by mathematician Luigi Fantappiè, syntropy helps explain how complex structures can emerge and evolve despite the overall entropic direction of the cosmos.
Entropy increases when energy disperses and becomes less available for work. According to the second law of thermodynamics, closed systems inevitably move toward states of maximum entropy—maximum disorder and minimum available energy. Yet throughout the universe, we observe systems that seem to defy this tendency, developing increasingly complex and ordered structures over time.
Syntropy describes this counter-entropic process—the local decrease in entropy that occurs when systems capture energy flows and use them to build and maintain complex structures. Rather than contradicting the second law, syntropy works within its constraints by creating local pockets of increasing order while exporting entropy to the surrounding environment.
Living systems exemplify this process. Plants capture sunlight and use its energy to organize simple molecules into complex carbohydrates, proteins, and other biological structures. Animals consume these energy-rich compounds and maintain their highly ordered bodies by continuously rebuilding tissues and regulating internal conditions. In both cases, the organisms maintain low internal entropy by exporting heat and waste products—higher entropy—to their surroundings.
This pattern extends beyond biology. Stars organize diffuse hydrogen into denser, more structured forms through gravitational attraction and nuclear fusion, releasing entropy as radiation. Planets develop complex atmospheric and geological systems through energy flows from their stars and internal heat. Human societies create increasingly complex technological and social structures by harnessing energy from various sources.
Several key principles characterize syntropic processes:
Energy Gradients: Syntropy requires energy flowing from high-concentration sources to lower-concentration sinks. These gradients provide the potential energy that systems can capture and use for organization.
Dissipative Structures: As physicist Ilya Prigogine demonstrated, systems far from thermodynamic equilibrium can spontaneously develop complex structures that help dissipate energy gradients more efficiently. These "dissipative structures" include hurricanes, convection cells, and living organisms.
Information Capture: Syntropic systems accumulate and preserve information over time. DNA represents the archetypal example—a molecule that stores information about successful structures and processes, allowing complexity to build across generations.
Increasing Efficiency: Syntropic systems tend to evolve toward greater efficiency in energy capture and utilization. This efficiency often manifests as increasing complexity, with more specialized components working together in more intricate arrangements.
The mathematics of syntropy involves concepts from non-equilibrium thermodynamics and information theory. While entropy can be quantified as S = k log W (where S is entropy, k is Boltzmann's constant, and W is the number of possible microscopic states), syntropy involves the reduction of this value in local systems coupled to energy flows.
Information theory provides another mathematical approach through the concept of negative entropy or "negentropy." Claude Shannon's information entropy equation:
H = -∑ p(x) log p(x)
measures the uncertainty or disorder in a system. When this value decreases, information content increases—a mathematical expression of syntropy.
Consciousness may represent a highly developed syntropic process. The human brain, consuming about 20% of the body's energy while comprising only 2% of its mass, maintains an extraordinarily complex structure that processes and generates information. Consciousness itself might be understood as an information-organizing system that creates meaning and coherence from sensory data—a local reduction in entropy achieved through energy expenditure.
The Poia framework connects syntropy to consciousness's role in reality creation. By selecting specific potentials from the field of possibility, consciousness reduces uncertainty (entropy) in the system, creating more ordered and meaningful patterns than would emerge from random processes alone. This selection doesn't violate physical laws but works within them to guide systems toward particular low-entropy states.
This perspective suggests that consciousness evolved precisely because it enhances syntropy—it helps living systems create and maintain order more effectively than unconscious processes alone. The capacity to anticipate, plan, and intentionally shape circumstances provides a powerful advantage in the ongoing effort to maintain low-entropy states in a high-entropy universe.
Syntropy thus offers a scientific framework for understanding how complexity increases throughout cosmic evolution without contradicting fundamental physical laws. It suggests that the emergence of consciousness represents not an anomaly but a natural development in the universe's capacity to generate local order within the larger context of increasing entropy.
Consciousness as an Emergent Property
Consciousness—that mysterious first-person experience of awareness—may represent the most profound example of emergence in the known universe. Rather than existing as a fundamental substance or property, consciousness might emerge from the complex interactions of simpler components that individually display no awareness.
This emergent perspective offers a middle path between reductionist materialism (which struggles to explain subjective experience) and dualism (which struggles to explain mind-body interaction). Consciousness emerges from physical processes but possesses properties and causal powers not reducible to those processes—just as wetness emerges from water molecules without being a property of individual molecules.
Several characteristics suggest consciousness's emergent nature:
Level-Specific Properties: Consciousness displays qualities not present in its neural components. Individual neurons don't experience qualia (subjective sensations), yet the system they form somehow generates the experience of colors, sounds, emotions, and thoughts.
Holistic Function: Consciousness operates as an integrated whole rather than a collection of separate processes. This integration creates the unified field of experience where diverse sensations, thoughts, and feelings appear within a single awareness.
Downward Causation: While emerging from neural activity, consciousness appears to influence that activity through attention, intention, and decision-making. This bidirectional causality characterizes complex emergent systems.
Threshold Effects: Consciousness seems to require a critical level of complexity and integration before emerging. Simple neural systems don't generate it, suggesting consciousness appears only when certain organizational thresholds are crossed.
The neuroscientific evidence increasingly supports this emergent view. Giulio Tononi's Integrated Information Theory proposes that consciousness emerges when a system integrates information in ways that create a whole greater than the sum of its parts. The theory quantifies this integration through a value called phi (Φ), which measures how much information a system contains beyond the information in its individual components.
Similarly, the Global Neuronal Workspace theory suggests consciousness emerges when specialized brain modules share information through a central network accessible to multiple processes. This sharing creates a global availability that transforms localized neural processing into conscious experience.
These theories align with the broader understanding of emergence in complex systems. Just as temperature emerges from molecular motion without being a property of individual molecules, consciousness might emerge from neural activity without being a property of individual neurons. The pattern of organization matters as much as the components themselves.
This emergent perspective helps resolve several philosophical puzzles about consciousness:
The Hard Problem: While not eliminating the mystery of why physical processes generate subjective experience, emergence clarifies that we shouldn't expect to find consciousness in fundamental particles or forces. It exists at a specific level of organization, emerging only when certain complex patterns form.
Mind-Body Interaction: If consciousness emerges from neural activity while also influencing it, the apparent causal gap between mental and physical domains closes. Mind and brain interact not as separate substances but as different levels of description of the same complex system.
Evolution of Consciousness: Emergence explains how consciousness could evolve gradually rather than appearing suddenly. As neural systems developed greater complexity and integration, consciousness would emerge in increasingly sophisticated forms, providing adaptive advantages through enhanced information processing.
The Poia framework extends this emergent understanding by suggesting consciousness not only emerges from physical complexity but participates in reality's ongoing creation. As an emergent property, consciousness gains the capacity to influence which potentials manifest from the quantum field—not by violating physical laws but by operating within them at a higher level of organization.
This perspective positions consciousness as neither an epiphenomenon (a passive byproduct of physical processes) nor a supernatural force (operating outside physical laws). Instead, consciousness represents nature's capacity to generate increasingly complex forms of organization that achieve new causal powers through emergence.
The emergence of consciousness may represent a natural stage in cosmic evolution—a development as significant as the emergence of life itself. Just as life transformed matter into self-replicating, adaptive systems, consciousness transforms life into self-aware, intentional systems capable of understanding and influencing their own development. This represents not a break with physical processes but their continuation into new domains of complexity.
The Evolutionary Advantage of Consciousness
If consciousness emerges from complex neural systems, what evolutionary advantage does it provide? Why would natural selection favor the development of subjective awareness rather than just sophisticated unconscious processing? This question touches the heart of why consciousness exists at all.
Several compelling explanations suggest how consciousness provides adaptive benefits:
Integrated Information Processing: Consciousness creates a unified workspace where diverse information can be combined in novel ways. Rather than processing sensory inputs through separate, specialized modules, conscious awareness allows information from different domains to interact—combining visual cues with memories, emotional responses with logical reasoning, or social knowledge with physical perception. This integration enables more sophisticated responses to complex situations.
Counterfactual Simulation: Consciousness permits mental simulation of potential actions and their outcomes without requiring physical trial and error. This capacity to imagine "what might happen if..." provides enormous adaptive advantages, allowing organisms to avoid dangers and pursue opportunities based on internal modeling rather than actual experience. The ability to learn from imagined scenarios rather than just lived experiences accelerates adaptation.
Flexible Response Selection: While unconscious processes excel at rapid, stereotyped responses to familiar situations, consciousness enables flexible, context-sensitive responses to novel challenges. This adaptability proves particularly valuable in complex, changing environments where pre-programmed reactions would prove insufficient.
Social Coordination: Consciousness facilitates sophisticated social interaction by enabling the modeling of other minds ("theory of mind"). The ability to understand others' perspectives, intentions, and knowledge states allows for more effective cooperation, communication, and competition—crucial advantages for social species like humans.
Extended Temporal Integration: Conscious awareness connects past experiences with present circumstances and anticipated futures, creating a temporally extended sense of self. This temporal integration enables long-term planning, delayed gratification, and the accumulation of knowledge across time—all significant evolutionary advantages.
These benefits help explain why consciousness would emerge through natural selection despite its apparent metabolic costs. The human brain consumes approximately 20% of the body's energy while comprising only 2% of its mass. This high energy investment must provide compensatory advantages, and the capabilities enabled by consciousness offer compelling candidates.
The Poia framework extends this evolutionary understanding by suggesting consciousness provides another crucial advantage: it allows organisms to participate more directly in selecting which potentials manifest from the quantum field. If reality exists as a field of possibilities until observed, then conscious observation represents a powerful capacity to influence which possibilities become actual.
This selective function would provide significant advantages. Organisms capable of directing attention and intention effectively could bias probability distributions toward favorable outcomes—not through supernatural intervention but through natural principles of quantum selection. This capacity would complement other cognitive advantages, enhancing an organism's ability to navigate complex environments successfully.
From this perspective, consciousness evolved not just as a sophisticated information processing system but as an interface between organisms and the field of potential. The ability to collapse quantum possibilities into specific actualities through observation and intention would provide a powerful tool for survival and reproduction, especially in complex, unpredictable environments.
This understanding helps explain why consciousness feels like something rather than nothing—why it has subjective qualities rather than operating as a purely functional process. The phenomenal aspects of consciousness—the felt qualities of experience—may be intrinsically connected to its role in selecting potentials from the quantum field. The "what it feels like" aspect of consciousness might be inseparable from its reality-selecting function.
The evolution of consciousness thus represents not just an enhancement of information processing but a fundamental shift in how organisms relate to reality. Rather than merely responding to a fixed external world, conscious beings participate in creating the reality they experience through the selective power of awareness and intention. This participatory relationship with reality may represent consciousness's most significant evolutionary advantage.
Complexity and the Evolution of Consciousness
The evolution of consciousness follows a trajectory of increasing complexity, from simple awareness in primitive organisms to the rich self-reflective consciousness of humans. This developmental path reveals how consciousness expands its capabilities through greater neural complexity, information integration, and organizational sophistication.
The earliest forms of consciousness likely emerged as simple awareness of environmental stimuli—the capacity to detect and respond to light, chemicals, temperature, or pressure. Single-celled organisms like paramecia display primitive stimulus-response behaviors that might represent the most basic form of awareness—a rudimentary capacity to distinguish self from environment and respond accordingly.
As multicellular organisms evolved more specialized sensory systems and neural networks, consciousness likely developed greater differentiation and integration. Simple nervous systems allowed for more sophisticated detection of environmental features and coordination of responses. The appearance of centralized nervous systems with primitive brains marked a significant advance, enabling more complex forms of perception and behavior.
The evolution of vertebrate brains brought further developments in consciousness. The emergence of the limbic system added emotional dimensions to awareness, while the expansion of the cerebral cortex enabled more sophisticated sensory processing, memory, and eventually abstract thought. Each advance in neural complexity supported new capabilities in conscious experience.
This evolutionary progression follows several key principles:
Increasing Integration: More advanced forms of consciousness integrate information across more domains—combining multiple sensory channels, emotional responses, memories, and conceptual understanding into unified experiences.
Greater Temporal Depth: Consciousness evolves to encompass longer time horizons—from immediate stimulus-response in simple organisms to the human capacity for autobiographical memory and long-term planning.
Enhanced Self-Reference: More complex consciousness includes increasingly sophisticated self-models—from basic body awareness to the human capacity for introspection and self-reflection.
Expanded Abstraction: Advanced consciousness can represent increasingly abstract concepts and relationships, moving beyond concrete sensory experience to understand patterns, categories, and principles.
Heightened Intentionality: Complex consciousness directs attention and intention with greater precision and flexibility, focusing awareness selectively rather than responding automatically to stimuli.
These developments reflect broader patterns in the evolution of complexity. As systems develop more specialized components and more intricate connections between them, new emergent properties appear. Each level of organization builds upon previous levels while introducing novel capabilities that couldn't be predicted from simpler components.
The Poia framework suggests this evolution serves a profound purpose: as consciousness develops greater complexity, it becomes more effective at bridging potential and actuality. Simple awareness might collapse quantum possibilities in basic ways, but complex consciousness can select potentials with greater precision and intentionality. The evolution of consciousness thus represents the universe developing increasingly sophisticated means of manifesting specific potentials from the field of possibility.
This perspective aligns with what philosopher Pierre Teilhard de Chardin called the "complexification" of the cosmos—a tendency toward increasing complexity and consciousness throughout evolution. From this viewpoint, the emergence of human consciousness represents not an accident but a natural development in the universe's capacity for self-awareness and intentional creation.
The future evolution of consciousness might continue this trajectory. Technologies that enhance neural integration, expand sensory capabilities, or facilitate collective intelligence could potentially support new emergent forms of awareness. Similarly, practices that develop attention, self-awareness, and intentionality might unlock latent capacities within existing neural structures.
This evolutionary perspective suggests consciousness isn't static but continues to develop through both biological and cultural processes. The human capacity for self-directed evolution—our ability to shape our own development through technology, education, and contemplative practices—may represent a new phase in consciousness evolution, where awareness begins to guide its own emergence rather than being shaped solely by natural selection.
Understanding consciousness as an evolving emergent property helps us appreciate both its biological foundations and its potential for further development. Rather than seeing consciousness as either a mysterious anomaly or a mere epiphenomenon, we can recognize it as a natural expression of complexity—the universe's capacity to generate increasingly sophisticated forms of awareness through self-organization.
Collective Consciousness and Social Complexity
Just as individual consciousness emerges from networks of neurons, forms of collective consciousness can emerge from networks of individuals. Social systems—from small groups to global civilization—develop emergent properties that transcend individual awareness while arising from the interactions between conscious beings.
These collective phenomena don't require mystical explanations. They emerge through the same principles that generate complexity in other systems—feedback loops, self-organization, and emergence. When individuals communicate and coordinate their actions, patterns appear at the group level that wouldn't exist without this interaction.
Several forms of collective consciousness can be identified:
Cultural Knowledge Systems: Shared beliefs, values, languages, and practices that exist beyond any individual mind. These systems shape individual consciousness while being shaped by it, creating feedback loops that evolve over generations.
Social Synchronization: The tendency for groups to align their behaviors, emotions, and even physiological states through interaction. Examples include audience applause spontaneously synchronizing or emotional contagion spreading through crowds.
Distributed Cognition: Problem-solving capacities that emerge when individuals combine their cognitive resources. Complex challenges that exceed individual capabilities can be addressed through collaborative thinking and specialized roles.
Group Awareness: The capacity of teams or communities to develop shared attention, common goals, and collective identity. This shared focus enables coordinated action toward purposes no individual could accomplish alone.
These collective phenomena display many characteristics of complex adaptive systems. They self-organize without central control, develop emergent properties not present in individuals, maintain themselves through feedback loops, and evolve over time in response to changing conditions.
The mathematics of network theory helps explain how these collective properties emerge. Concepts like small-world networks (where most nodes connect to nearby neighbors with occasional long-distance connections) and scale-free networks (where connection patterns follow power laws) describe how information flows through social systems. These network structures enable both local specialization and global integration—a balance that characterizes complex consciousness at both individual and collective levels.
The development of collective consciousness follows an evolutionary trajectory similar to individual consciousness. Simple forms appear in small groups through direct interaction. More complex forms emerge with the development of symbolic communication, especially writing, which allows knowledge to accumulate across generations. Digital technologies enable new forms of collective awareness by connecting minds across space and time with unprecedented speed and scale.
The Poia framework suggests these collective forms of consciousness might influence reality creation in powerful ways. If individual consciousness can select which potentials manifest from the quantum field, collective consciousness might exert even stronger selective pressure through coherent attention and intention across many minds. This perspective helps explain phenomena like cultural paradigms that shape what members of a society can perceive and create.
This understanding has practical implications. Practices that enhance collective coherence—from group meditation to collaborative visioning to aligned organizational cultures—might increase a group's capacity to manifest intended outcomes. Conversely, social fragmentation and conflict might reduce collective creative power by generating incoherent or contradictory intentions.
The evolution of collective consciousness may represent a crucial frontier in human development. As global challenges like climate change, technological disruption, and social inequality exceed the problem-solving capacity of individual minds or fragmented groups, more sophisticated forms of collective awareness become increasingly necessary. Technologies and practices that enhance our capacity for coherent collective thinking and action may prove essential for addressing these complex challenges.
This perspective doesn't diminish individual consciousness but contextualizes it within broader fields of awareness. Just as neurons maintain their distinct functions while participating in the emergent phenomenon of mind, individuals maintain their unique perspectives while contributing to collective forms of consciousness. The relationship is reciprocal—individual awareness shapes collective patterns, while collective patterns influence individual experience.
Understanding consciousness as both individual and collective helps resolve the apparent tension between personal autonomy and social connection. Rather than seeing these as opposing forces, we can recognize them as complementary aspects of consciousness operating at different levels of complexity. The most developed consciousness might integrate both individual uniqueness and collective participation—maintaining a distinct perspective while recognizing its embeddedness in larger fields of awareness.
Practical Applications: Working with Complexity
The principles of complexity and emergence offer practical approaches for working with both individual and collective consciousness. Rather than trying to control complex systems through linear, reductionist methods, these approaches leverage natural tendencies toward self-organization and emergence.
Several key strategies emerge from complexity thinking:
Work with Patterns, Not Just Parts: Instead of focusing exclusively on individual components, identify and influence the patterns of interaction between them. In personal development, this means attending to relationships between thoughts, emotions, and behaviors rather than addressing each in isolation. In social change, it means focusing on communication networks and feedback loops rather than just individual behaviors.
Leverage Small Changes at Critical Points: Complex systems often contain leverage points where small inputs can produce large effects. Identifying these points—whether they're beliefs that shape many behaviors, hub individuals in social networks, or critical thresholds in system parameters—allows for efficient intervention with minimal resources.
Create Enabling Conditions Rather Than Forcing Outcomes: Rather than trying to directly control complex systems (which often creates resistance or unintended consequences), establish conditions that enable desired patterns to emerge naturally. This might involve changing incentives, providing resources, removing barriers, or introducing catalysts that facilitate self-organization.
Maintain Adaptive Tension: Creativity and evolution occur when systems exist in a state of productive tension—not so comfortable that they stagnate nor so stressed that they break down. Maintaining this "sweet spot" of adaptive tension helps systems develop new capabilities while preserving essential functions.
Use Feedback for Learning, Not Just Control: Rather than using feedback solely to correct deviations from predetermined goals, use it as information that helps the system learn and evolve. This approach treats unexpected outcomes not as failures but as valuable data that reveals the system's actual patterns and potentials.
These strategies apply across domains, from personal consciousness to organizational development to social change:
In personal development, they suggest approaches like:
- Cultivating awareness of thought patterns rather than fighting individual thoughts
- Identifying core beliefs that influence many aspects of experience
- Creating environments that support desired states of consciousness
- Maintaining productive challenge without overwhelming stress
- Using mindfulness to learn from all experiences rather than judging them
In organizational contexts, they translate to practices like:
- Focusing on communication patterns and cultural norms rather than just individual performance
- Identifying informal leaders and network hubs who influence collective behavior
- Designing spaces and processes that enable collaboration and innovation
- Balancing stability and change to maintain adaptive capacity
- Using failures and successes equally as learning opportunities
In social change efforts, they suggest strategies such as:
- Mapping influence networks to understand how behaviors and beliefs spread
- Identifying tipping points where social norms might shift rapidly
- Creating platforms and infrastructures that enable self-organized action
- Maintaining tension between current reality and vision without generating despair
- Treating unexpected outcomes as information about the system rather than as failures
The Poia framework adds another dimension to these approaches by recognizing consciousness as an active force in reality creation. This suggests additional strategies:
Coherent Intention: Developing practices that align thoughts, emotions, beliefs, and actions to create coherent intentional fields that more effectively influence probability distributions in the quantum field.
Collective Resonance: Cultivating shared awareness and aligned purpose in groups to amplify their collective influence on potential outcomes through resonant intention.
Attentional Discipline: Training the capacity to direct and sustain attention precisely, recognizing that where awareness focuses helps determine which potentials manifest from the field of possibility.
Field Sensitivity: Developing the ability to sense subtle patterns and potentials in complex systems before they fully manifest, allowing more timely and effective intervention.
These approaches don't promise complete control over complex systems—an impossible goal given their inherent unpredictability. Instead, they offer ways to participate more skillfully in complexity, influencing patterns and probabilities while remaining adaptable to emerging developments.
This perspective transforms how we approach challenges at all scales. Rather than imposing predetermined solutions through force, we can work with the natural tendencies of complex systems—including consciousness itself—to facilitate the emergence of new patterns and possibilities. This approach combines humility about our ability to control complexity with confidence in our capacity to influence it through awareness, intention, and skillful action.
Conclusion: Complexity, Consciousness, and Creation
The emergence of complexity throughout the universe—from subatomic particles organizing into atoms, atoms into molecules, molecules into living cells, cells into organisms, and organisms into societies—reveals a fundamental creative principle at work in nature. Far from being a universe of random chaos or rigid determinism, we inhabit a cosmos that continuously generates new forms of order and organization through the principles of emergence and self-organization.
Consciousness represents perhaps the most remarkable expression of this creative principle—an emergent phenomenon that arises from complex neural systems yet transcends them through unique capacities for self-awareness, intention, and meaning-making. Rather than standing apart from natural processes, consciousness exemplifies nature's tendency to evolve increasingly complex forms of organization that develop novel properties and causal powers.
The Poia framework suggests this emergence of consciousness serves a profound purpose: it enables the universe to know itself and participate in its own creation. Through conscious observation and intention, potential patterns from the quantum field collapse into actual experiences, creating a participatory reality where mind and matter engage in continuous creative dialogue. Consciousness doesn't just witness reality but helps shape which potentials manifest from the field of possibility.
This perspective resolves several longstanding paradoxes:
The Mind-Body Problem: Rather than treating mind and matter as fundamentally different substances that somehow interact, complexity theory reveals how consciousness emerges from physical processes while developing causal powers that influence those processes—a bidirectional relationship between different levels of organization rather than different kinds of substance.
Order and Entropy: The apparent contradiction between increasing entropy (disorder) and the evolution of complex systems resolves when we recognize how open systems can decrease their internal entropy by exporting it to their environment. Life and consciousness represent sophisticated examples of this process—creating local pockets of increasing order within the larger context of universal entropy increase.
Determinism and Free Will: The tension between physical causality and conscious choice finds resolution in complexity theory's concept of downward causation—how emergent patterns constrain and guide the behavior of their components without violating physical laws. Consciousness influences neural activity not by overriding physical causality but by operating as a higher-level pattern within it.
Science and Spirituality: The traditional separation between objective science (studying the physical world) and subjective spirituality (exploring consciousness) dissolves when we recognize consciousness as an emergent natural phenomenon that actively participates in reality creation. Both domains address aspects of the same unified process—the universe evolving toward greater complexity and self-awareness.
This unified understanding suggests a profound vision of cosmic purpose—not imposed from outside but emerging from within the creative processes of nature itself. The universe appears to be evolving toward increasing complexity, consciousness, and creative participation. Each new level of organization—from atoms to molecules to cells to organisms to minds—represents a step in this evolution, with consciousness enabling more direct participation in selecting which potentials become actual.
This doesn't mean the universe has reached its final form. The principles of complexity and emergence suggest continued evolution toward even more sophisticated forms of organization and awareness. Just as individual consciousness emerged from neural complexity, new forms of collective consciousness may be emerging from the increasing interconnection of human minds through culture and technology. These collective forms might develop capacities for reality creation that transcend individual capabilities while incorporating them.
The practical implication of this understanding is profound: we are not passive observers but active participants in reality's unfolding. Through our awareness, intention, and action, we contribute to determining which potentials manifest from the field of possibility. This participation carries both opportunity and responsibility—the chance to consciously shape our experience and the obligation to consider the broader consequences of our creative influence.
By understanding the principles of complexity and emergence, we can participate more skillfully in this creative process. Rather than imposing rigid control or surrendering to chaos, we can work with the natural tendencies of complex systems—including consciousness itself—to facilitate the emergence of patterns that enhance life, awareness, and meaning. This approach combines scientific understanding with spiritual wisdom, recognizing consciousness as both a natural phenomenon and a creative force in reality's ongoing evolution.
The emergence of complexity thus reveals a universe of extraordinary creativity—one that continuously generates new forms of order, organization, and awareness through natural principles rather than external design. Consciousness represents not an anomaly in this creative process but its most sophisticated expression—the universe evolved to know itself and participate in its own creation. Understanding this participatory relationship transforms how we perceive our place in the cosmos and how we approach the art of conscious living.